Introduction
In the modern world, the application of laser technology has permeated various fields including medicine, communications, manufacturing, and defense. Central to the function of lasers is the mechanism through which they generate beams of coherent light. This process is fascinatingly orchestrated within the heart of crystals. Through this discourse, we unravel the layers of science that make this possible.
Understanding Lasers
Understanding the core operations and characteristics of lasers is pivotal to appreciating their extensive applications in modern life. The term “laser” stands for Light Amplification by Stimulated Emission of Radiation, which succinctly encapsulates the foundational principle on which lasers operate. This principle revolves around the behavior of atoms when subjected to external energy. As atoms absorb this energy, they get excited to a higher energy state. Upon returning to their original or ground state, these atoms emit photons, which are particles of light. This emission of photons is what gives birth to the laser beam. However, what differentiates laser light from ordinary light is its unique characteristics.
Laser light is known for being coherent, monochromatic, and highly collimated, characteristics that are crucial for the laser’s performance in various applications. Coherence refers to the phase relationship between the waves in light. In a laser beam, the light waves are in phase with each other over a long distance, which means they oscillate in harmony, maintaining a fixed phase relationship. This coherence allows for the light to stay focused over a long distance, providing high-intensity light that’s crucial for applications demanding precision.
On the other hand, monochromaticity refers to the single wavelength or color of the laser light. Unlike white light, which is a mix of many colors or wavelengths, laser light consists of one color, meaning that all the photons in a laser beam have the same energy level, and hence, same wavelength. This singular wavelength is what makes laser light highly controllable and precise, which is indispensable in scenarios such as scientific research or medical surgeries where a specific wavelength of light is required.
The collimation of laser light is another vital characteristic, referring to the parallelism of light rays within the beam. A highly collimated laser beam barely diverges even over long distances, thus maintaining a tight beam that is essential for tasks requiring pinpoint accuracy. The near-parallel rays of laser light ensure that the beam does not spread out and lose its intensity, making lasers an invaluable tool in numerous fields like manufacturing, medicine, communications, and many more, where precision and control are paramount.
In essence, understanding the basic operational mechanics and the unique characteristics of laser light paves the way for a deeper appreciation of the power and potential of laser technology. Through the fundamental process of excitation and emission of photons from atoms, paired with the laser light’s coherence, monochromaticity, and collimation, lasers stand as a significant pillar of modern technological advancements, opening doors to endless possibilities and applications.
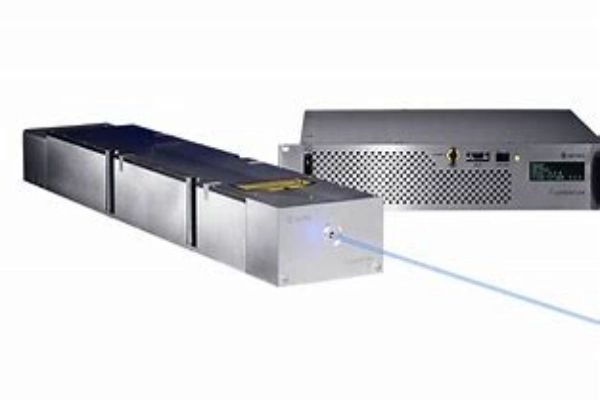
Crystals as the Heartbeat of Lasers
Crystals indeed serve as the heartbeat of lasers, embodying the core mechanism that enables the fascinating phenomenon of light amplification. Their unique structural attributes provide the perfect milieu for the orchestration of controlled interactions between light and matter. At the heart of laser technology lies the process of amplifying light through the stimulated emission of radiation, a process elegantly hosted within the lattice of crystals. Their orderly atomic structure creates a conducive environment for controlling the behavior of photons, the fundamental particles of light, thus playing a pivotal role in laser operation.
The journey of laser beam creation commences with the infusion of energy into the crystal. This energy jolts the atoms within the crystal into higher energy states, setting the stage for the subsequent drama of stimulated emission. As these excited atoms make their way back to their ground state, they emit photons, which then rally to form a coherent army of light, marching in unison to the beat of a laser beam.
Among the ensemble of crystals, some stars shine brighter in the realm of laser technology. The Ruby crystal, with its vibrant red allure, was the protagonist in the tale of the first-ever laser. Its ability to house and channel energy makes it a cherished choice in the laser community. Then, there’s the Nd:YAG (Neodymium-doped Yttrium Aluminum Garnet) crystal, a stalwart in the world of solid-state lasers, known for its robustness and versatility.
It stands tall, delivering high-powered performance across a myriad of applications, from industrial cutting and welding to medical surgeries. The Ti:Sapphire (Titanium-doped Sapphire) crystal is another gem, celebrated for its broad tunable range, allowing for the generation of laser beams of varying wavelengths. This feature is indispensable in scientific research where different wavelengths are employed to probe and understand the mysteries of nature.
The choice of crystal is not a mere whimsy but a calculated decision based on the intended application of the laser. The unique properties of these crystals, such as their thermal conductivity, damage threshold, and optical homogeneity, are meticulously evaluated to ensure they align with the requisites of the laser system in question. The harmony between the crystal and the surrounding laser apparatus is crucial for the successful amplification and control of light, thus underlining the indispensable role of crystals in laser technology.
In summation, the realm of laser technology is a grand theater where crystals play the leading role, orchestrating the ballet of photons that dance to the rhythm of stimulated emission. Their structural elegance coupled with their diverse optical properties, make crystals the cornerstone of laser technology, facilitating the generation of laser beams that continue to illuminate the path of human progress across various fields.
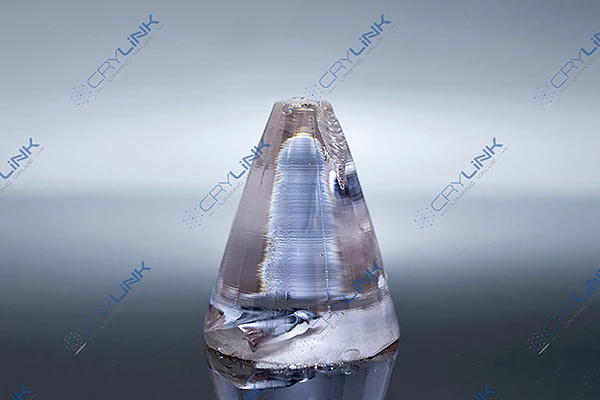
The Journey of Laser Production
The enthralling journey of laser production begins in the heart of a crystal, where the initial spark of energy sets off a cascade of events leading to the birth of a laser beam. The introduction of energy, typically executed by a flashlamp or another laser, serves as the catalyst, propelling the atoms within the crystal into higher energy states. This pivotal step, known as pumping, is the precursor to the magic that follows.
As these energized atoms hustle back to their more stable, ground state, they relinquish their extra energy in the form of photons. This phenomenon, dubbed stimulated emission, is the cornerstone of laser operation. Each emitted photon is a clone of the one that stimulated its emission, possessing the same energy, phase, and direction. This ensures the coherence of the light, a characteristic hallmark of lasers.
Now, within the orchestrated confines of a resonant cavity, the drama unfolds. The nascent photons find themselves in a hall of mirrors, bouncing back and forth between the reflective walls of the cavity. Each pass through the crystal triggers the emission of more photons from the remaining excited atoms, in a beautifully choreographed dance of light. This interplay, known as resonant cavity reflection, serves as the amplification stage of the journey, bolstering the intensity of the light with each reflection.
As the photons multiply, uniting in phase and direction, they form a formidable army of coherent light, marching forth as a robust laser beam ready to conquer whatever task lies ahead. The resonant cavity acts as the crucible, forging the light into a powerful laser beam through a meticulous process of reflection and amplification.
This journey, from the quiet halls of a crystal to the resonant echoes of a cavity, encapsulates the elegance and precision of laser production. Through a well-choreographed ballet of energy transitions and photon reflections, the laser emerges as a beacon of coherent, monochromatic light, poised to cut through steel, mend flesh, or carry data across the void. The meticulous process of laser production showcases the marvel of physics and engineering, as the mundane is transmuted into the miraculous, one photon at a time.
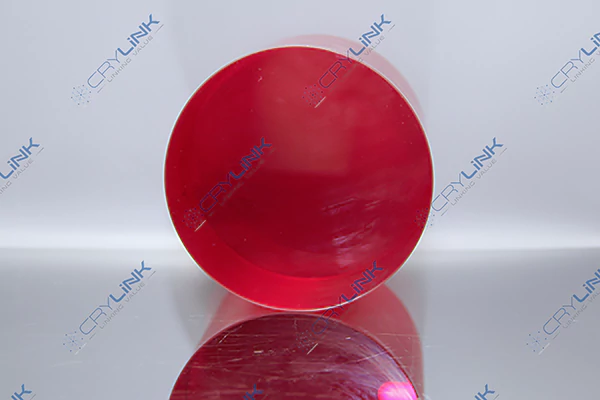
Tuning and Modulating Laser Beams
The art of tuning and modulating laser beams is akin to orchestrating a symphony, where each adjustment results in a harmonized output tailored for specific applications. The conductor’s baton in this symphony is the various techniques like wavelength tuning, Q-switching, and mode locking that fine-tune the laser’s characteristics to meet the demands of a myriad of tasks.
The act of wavelength tuning is a fine adjustment that tweaks the color of the laser beam by modifying the properties of the crystal or the resonant cavity. This is akin to choosing the right musical note for a melody, where each wavelength corresponds to a unique color and energy. This tuning is vital for ensuring the laser beam is perfectly pitched for its intended application, be it slicing through metal in a factory, correcting vision in a surgical suite, or probing the mysteries of the universe in a research lab.
On the other hand, Q-switching and mode locking are sophisticated techniques that modulate the laser’s properties, ensuring they strike the right chord for specific tasks. Q-switching is like a dramatic pause in a musical piece, building up energy within the laser medium before releasing it in a powerful burst of light. This technique is crucial for applications demanding high-powered laser pulses. Mode locking, in contrast, synchronizes the laser’s different modes into a coherent pulse, akin to harmonizing the different sections of an orchestra into a unified melody. This synchronization is critical for producing ultra-short laser pulses used in a variety of precise applications.
The stage where this laser symphony is performed is vast, with crystal-based lasers finding their spotlight in a plethora of fields. In the medical arena, they execute surgical procedures with precision, while in the industrial sector, they cut and weld materials with finesse. In the realm of telecommunications, these lasers transmit data over vast distances at the speed of light, orchestrating the flow of information that is vital in the modern world.
In essence, the tuning and modulating of laser beams, facilitated by sophisticated techniques and crystal-based laser technology, enable the precise control and customization of lasers. This, in turn, opens up a vast spectrum of applications, showcasing the versatility and indispensability of laser technology in advancing human endeavor across various fields. Each tweak and modulation in the laser’s properties is a note in the grand symphony of laser applications, contributing to the melody of innovation and progress that resonates through the corridors of modern technology.
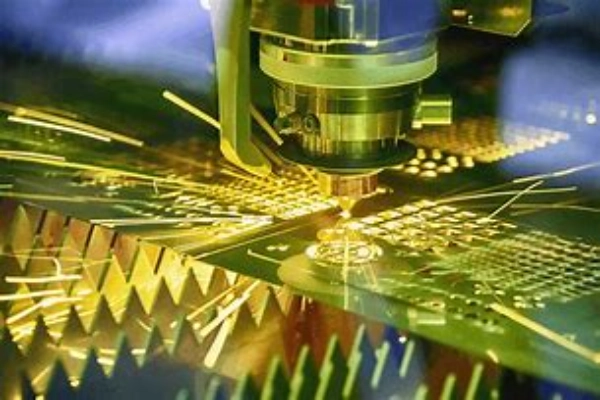
Conclusion
The science of producing laser beams with crystals is a meticulous blend of physics and engineering. The journey from pumping energy into a crystal to finally achieving a highly coherent, monochromatic beam of light is a testament to the advancements in laser technology. With continuous research and development, the boundaries of what is achievable with crystal-based lasers continue to expand.
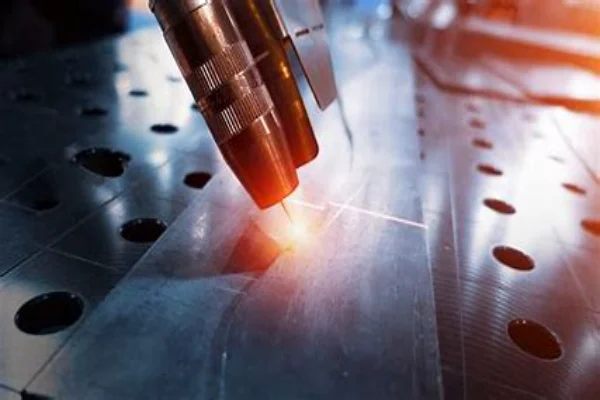
Frequently Asked Questions
- What types of crystals are used in laser technology?
- Commonly used crystals include Ruby, Nd:YAG, and Ti:Sapphire, each with unique properties conducive for laser production.
- How is energy pumped into the crystal?
- Energy is typically pumped into the crystal using a flashlamp or another laser, which excites the atoms within the crystal.
- What is stimulated emission?
- Stimulated emission is the process where excited atoms return to their ground state, emitting photons that contribute to the laser beam.
- How is the wavelength of a laser beam tuned?
- The wavelength can be tuned by adjusting the properties of the crystal or the resonant cavity, allowing for customization of the laser for various applications.
- What are some applications of crystal-based lasers?
- They find applications in medicine, industries for cutting and welding tasks, and telecommunications for data transmission.
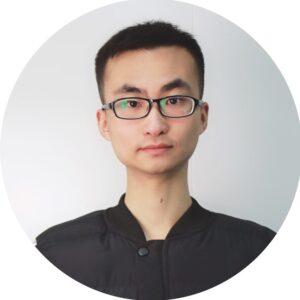
Frank
Frank graduated from the University of Shanghai for Science and Technology, majoring in optics. As a technical engineer at Crylink Company, he deeply understands crystal materials and laser components.
Related Video(s) with this Article
Related Product(s) with this Article
Related Application(s) with this Article