1. Understanding BBO SHG
Introduction
Beta-barium borate (BBO) is a nonlinear optical crystal widely used in second harmonic generation (SHG) experiments due to its high nonlinear coefficient, wide transparency range, and excellent optical homogeneity. SHG is a nonlinear optical process in which two photons of the same frequency combine to produce a single photon of twice the frequency. BBO SHG is used in a wide range of applications, including microscopy, spectroscopy, and laser systems.
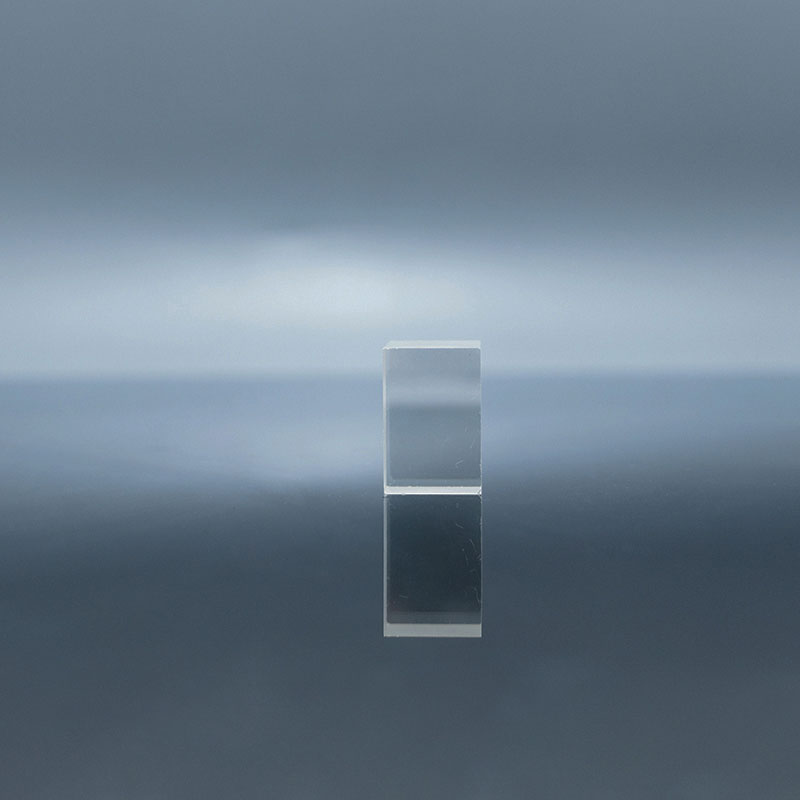
In this chapter, we will provide an introduction to BBO SHG, including its fundamental principles, advantages, and limitations. We will cover essential concepts such as nonlinear optics, phase matching, and birefringence, and explain how they relate to BBO SHG. The reader will learn how BBO SHG works, what factors affect its efficiency, and how to measure its performance. We will also provide a brief history of BBO SHG and its applications in various fields.
Fundamental principles of BBO SHG
BBO SHG is a second-order nonlinear optical process, which means that the intensity of the generated SHG signal is proportional to the square of the incident laser intensity. The process can be described by the following equation:
P2 = K P12 L,
where P1 is the incident laser power, P2 is the SHG power, L is the length of the crystal, and K is the nonlinear coefficient of the crystal. The nonlinear coefficient K depends on the crystal orientation, wavelength, and temperature.
The efficiency of BBO SHG is strongly dependent on phase matching, a condition in which the phase velocities of the fundamental and second harmonic waves are equal. Phase matching can be achieved by adjusting the crystal orientation, temperature, or angle of incidence of the laser beam. In addition, BBO SHG is affected by birefringence, a property of the crystal that causes the refractive index to vary with the polarization direction of the incident light.
Advantages of BBO SHG
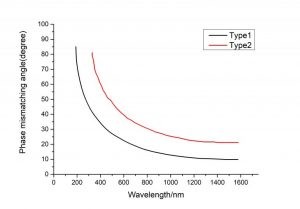
BBO SHG has several advantages over other nonlinear optical crystals. First, BBO has a high nonlinear coefficient, which means that it can produce a strong SHG signal with low incident power. Second, BBO has a wide transparency range, which allows it to be used with a variety of laser sources. Third, BBO has excellent optical homogeneity, which reduces optical distortion and improves signal quality. Fourth, BBO is relatively easy to grow and process, which makes it a cost-effective option for many applications.
Limitations of BBO SHG
Despite its many advantages, BBO SHG has some limitations that must be considered. First, BBO is a hygroscopic material, which means that it can absorb moisture from the environment and degrade over time. Second, BBO is susceptible to photorefractive damage, which can occur when the laser power is too high or when the crystal is exposed to prolonged laser irradiation. Third, BBO is birefringent, which can cause polarization-dependent effects in the SHG signal.
Applications of BBO SHG
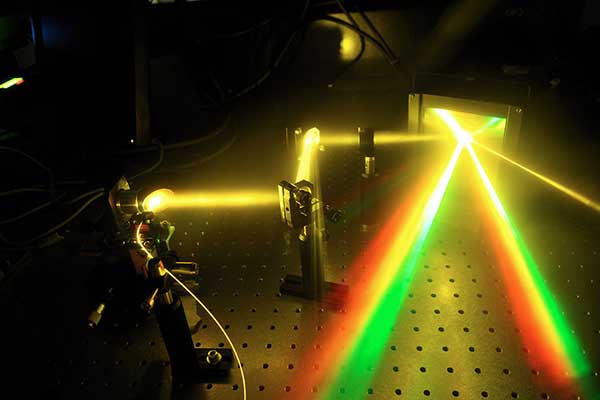
BBO SHG is used in a wide range of applications, including microscopy, spectroscopy, and laser systems. In microscopy, BBO SHG is used to image biological samples, such as collagen fibers and muscle tissue. In spectroscopy, BBO SHG is used to study the properties of materials, such as the bandgap energy of semiconductors. In laser systems, BBO SHG is used to generate high-power green laser beams for industrial and medical applications.
Conclusion
In this chapter, we have provided an introduction to BBO SHG, including its fundamental principles, advantages, and limitations. We have covered essential concepts such as nonlinear optics, phase matching, and birefringence, and explained how they relate to BBO SHG. The reader has learned how BBO SHG works, what factors affect its efficiency, and how to measure its performance. Additionally, the chapter provided a brief history of BBO SHG and its applications in various fields.
In summary, BBO SHG is a crucial tool in many areas of research and technology. Understanding the fundamental principles of BBO SHG, its advantages and limitations, and its applications is essential for optimizing experimental setups and obtaining high-quality results. The following chapters will provide detailed information on how to prepare BBO crystals, design experimental setups, and optimize performance for various applications.
2. BBO Crystal Growth and Processing
BBO crystals are essential components in second harmonic generation experiments. In this chapter, we will cover the essential aspects of BBO crystal growth and processing. The reader will learn how to prepare high-quality BBO crystals for SHG experiments, including the different techniques used for crystal growth, polishing, and cleaning. The chapter will also cover the common issues that can arise during BBO crystal processing and how to overcome them.
BBO Crystal Growth Techniques
There are several techniques available for growing BBO crystals, including the Czochralski method, the Bridgman method, and the flux method. The Czochralski method is the most commonly used technique for growing BBO crystals. This method involves melting the raw BBO material in a crucible and slowly pulling a seed crystal out of the melt. The Bridgman method is similar to the Czochralski method but involves moving the seed crystal through a temperature gradient to promote crystal growth. The flux method involves dissolving the raw BBO material in a flux material and slowly cooling the solution to promote crystal growth.
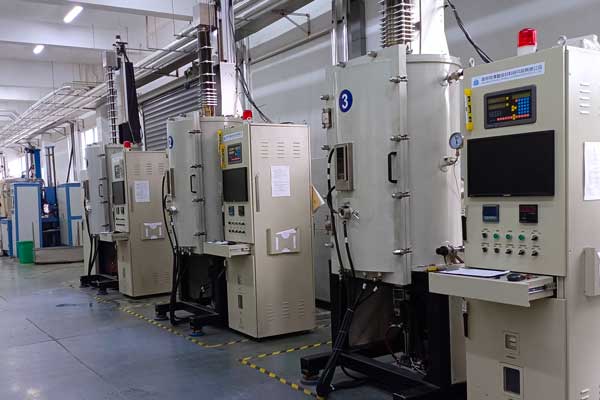
Polishing and Cleaning BBO Crystals
After growing BBO crystals, they must be polished and cleaned before use in SHG experiments. The polishing process involves grinding the crystal to a specific thickness and smoothing the surface to reduce scattering and improve transmission. The polishing process must be carefully controlled to avoid introducing surface defects that can affect the crystal’s performance.
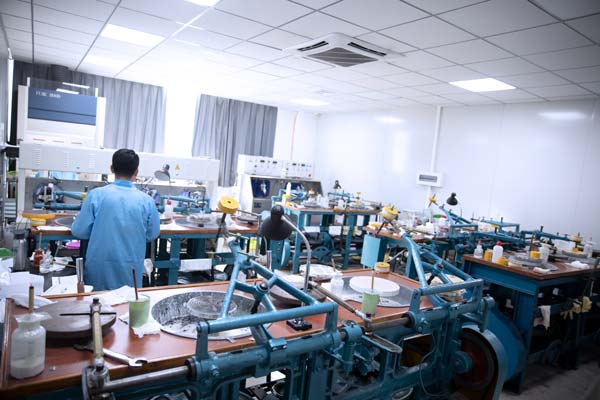
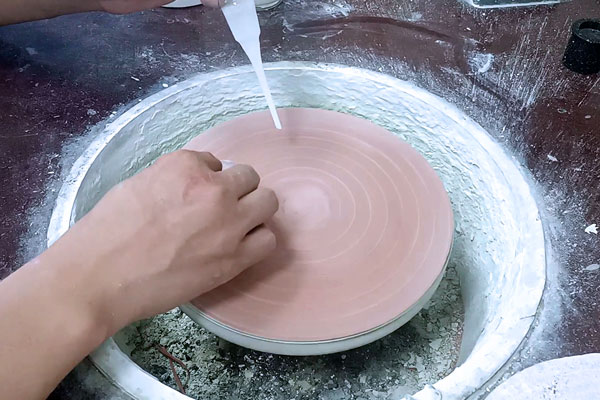
Once polished, BBO crystals must be thoroughly cleaned to remove any contaminants or residues that can affect their optical properties. The cleaning process usually involves soaking the crystal in a solvent, such as acetone or isopropanol, and then rinsing it with deionized water. Ultrasonic cleaning can also be used to remove stubborn contaminants. Care must be taken to avoid scratching or damaging the crystal during cleaning.
Common Issues in BBO Crystal Processing
Several issues can arise during BBO crystal processing that can affect the crystal’s performance. One common issue is crystal cracking, which can occur during growth or processing. Cracks can reduce the crystal’s optical quality and cause it to fracture under stress. To prevent cracking, the crystal growth and processing conditions must be carefully controlled.
Another common issue is crystal defects, such as dislocations or inclusions, which can affect the crystal’s optical properties. Defects can be introduced during growth or processing and must be carefully minimized to ensure optimal performance.
Additionally, BBO crystals are hygroscopic, which means they can absorb moisture from the environment and degrade over time. To prevent moisture absorption, BBO crystals should be stored in a dry environment, and handling should be kept to a minimum.
Conclusion
In this chapter, we have covered the essential aspects of BBO crystal growth and processing. The reader has learned how to prepare high-quality BBO crystals for SHG experiments, including the different techniques used for crystal growth, polishing, and cleaning. The chapter has also covered the common issues that can arise during BBO crystal processing and how to overcome them. By understanding these techniques and issues, researchers can obtain high-quality BBO crystals and optimize their SHG experiments for optimal performance.
3. Designing Experimental Setups for BBO SHG
Designing an experimental setup is a critical step in performing BBO SHG experiments. The experimental setup must be carefully designed to ensure that the laser source, optics, and detectors are properly selected and optimized for optimal SHG efficiency. In this chapter, we will provide a detailed guide to designing experimental setups for BBO SHG. The reader will learn how to select the appropriate laser source, optics, and detectors for optimal performance. The chapter will cover essential concepts such as beam shaping, polarization control, and wavelength tuning. The reader will also learn how to optimize the experimental parameters for maximum SHG efficiency.
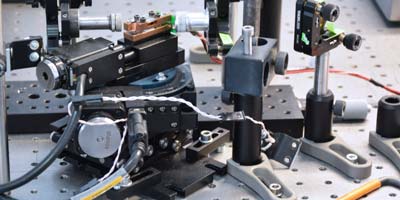
Laser Source
The laser source is one of the critical components of the experimental setup. The laser source must have sufficient power, stability, and coherence to generate a strong SHG signal. The most commonly used laser sources for BBO SHG experiments are Ti:sapphire lasers, Nd:YAG lasers, and frequency-doubled diode lasers.
Ti:sapphire lasers are widely used for their broad tuning range and high peak power. Nd:YAG lasers are popular due to their high repetition rates and reliability. Frequency-doubled diode lasers are cost-effective options for low-power applications.
Optics
Optics play a critical role in shaping the laser beam and controlling its polarization, which are essential for efficient SHG. The most commonly used optics in BBO SHG experiments are lenses, mirrors, and polarizers.
Lenses are used to focus the laser beam onto the BBO crystal and shape the beam profile. Mirrors are used to reflect and steer the laser beam, allowing it to be directed towards the crystal at the correct angle. Polarizers are used to control the polarization of the laser beam, which is crucial for achieving efficient SHG.
Beam Shaping
The laser beam shape can affect the efficiency of SHG. A well-shaped laser beam can maximize the overlap between the laser beam and the BBO crystal, leading to higher SHG efficiency. Beam shaping can be achieved using lenses or other optical elements to change the beam’s size, shape, and divergence.
Polarization Control
Controlling the polarization of the laser beam is crucial for efficient SHG. The polarization direction must be carefully aligned with the crystal axis to achieve phase matching. Polarization control can be achieved using polarizers or other optical elements, such as waveplates or birefringent crystals.
Wavelength Tuning
The wavelength of the laser source must be carefully tuned to achieve optimal SHG efficiency. The crystal’s nonlinear coefficient and phase matching conditions vary with the wavelength, and selecting the correct wavelength can improve SHG efficiency. Wavelength tuning can be achieved using a variety of techniques, including temperature tuning, angle tuning, or cavity length tuning.
Optimizing Experimental Parameters
Several experimental parameters can be optimized to improve SHG efficiency. These parameters include the laser power, crystal length, and crystal orientation. The laser power must be carefully controlled to avoid damage to the crystal and ensure optimal efficiency. The crystal length and orientation must be carefully selected to achieve phase matching conditions.
Conclusion
Designing an experimental setup is a critical step in performing BBO SHG experiments. The experimental setup must be carefully designed to ensure that the laser source, optics, and detectors are properly selected and optimized for optimal SHG efficiency. In this chapter, we have provided a detailed guide to designing experimental setups for BBO SHG. The reader has learned how to select the appropriate laser source, optics, and detectors for optimal performance.
The chapter has covered essential concepts such as beam shaping, polarization control, and wavelength tuning. The reader has also learned how to optimize the experimental parameters for maximum SHG efficiency. By understanding these techniques, researchers can optimize their experimental setups and achieve high-quality SHG results with BBO crystals. The next chapter will cover advanced techniques for improving SHG efficiency and reducing noise in BBO SHG experiments.
4. Advanced Techniques for Improving BBO SHG Efficiency
Improving the efficiency of BBO SHG experiments is essential for achieving high-quality results. In this chapter, we will cover advanced techniques for improving BBO SHG efficiency and reducing noise. The reader will learn how to use techniques such as quasi-phase matching, temperature tuning, and cavity-enhanced SHG to improve SHG efficiency. The chapter will also cover noise reduction techniques such as common-mode rejection and lock-in amplification.
Quasi-Phase Matching
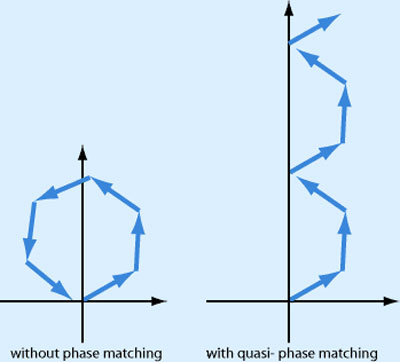
Quasi-phase matching (QPM) is a technique for achieving phase matching in BBO SHG experiments. QPM involves periodically varying the crystal’s domain structure to overcome the limitations of conventional phase matching. By varying the domain structure, the phase mismatch between the fundamental and SHG waves can be reduced, leading to higher SHG efficiency.
Temperature Tuning
Temperature tuning is a simple and effective technique for achieving phase matching in BBO SHG experiments. By adjusting the temperature of the crystal, the crystal’s nonlinear coefficient and phase matching conditions can be tuned to optimize SHG efficiency. Temperature tuning can be achieved using a temperature-controlled chamber or a Peltier cooler.
Cavity-Enhanced SHG
Cavity-enhanced SHG is a technique for enhancing the SHG efficiency by using a resonant cavity to enhance the fundamental and SHG waves’ interaction. By tuning the cavity length and the fundamental wavelength, the SHG signal can be significantly enhanced. Cavity-enhanced SHG is commonly used in high-power laser systems.
Common-Mode Rejection
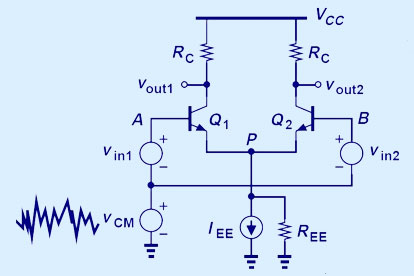
Common-mode rejection is a noise reduction technique that is commonly used in BBO SHG experiments. Common-mode noise can arise from various sources, including power fluctuations and laser intensity noise. By using balanced detectors, common-mode noise can be rejected, leading to higher signal-to-noise ratios.
Lock-In Amplification
Lock-in amplification is another noise reduction technique that is commonly used in BBO SHG experiments. Lock-in amplification involves modulating the laser power at a specific frequency and then detecting the SHG signal at the same frequency. By using a lock-in amplifier, noise from sources such as laser intensity fluctuations can be significantly reduced, leading to higher signal-to-noise ratios.
Conclusion
Improving the efficiency of BBO SHG experiments is essential for achieving high-quality results. In this chapter, we have covered advanced techniques for improving BBO SHG efficiency and reducing noise. The reader has learned how to use techniques such as quasi-phase matching, temperature tuning, and cavity-enhanced SHG to improve SHG efficiency. The chapter has also covered noise reduction techniques such as common-mode rejection and lock-in amplification. By understanding these advanced techniques, researchers can optimize their BBO SHG experiments and achieve high-quality results with reduced noise.
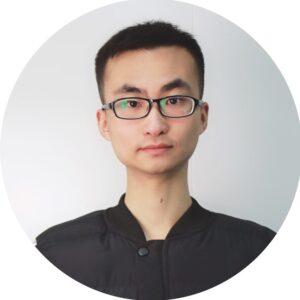
Frank
Frank graduated from the University of Shanghai for Science and Technology, majoring in optics. As a technical engineer at Crylink Company, he deeply understands crystal materials and laser components.
Related Video(s) with this Article
Related Product(s) with this Article
Related Application(s) with this Article