Introduction
In the realm of precision timekeeping, atomic clocks stand as the pinnacle of accuracy. These devices, which harness the predictable oscillations of atoms, have revolutionized our understanding of time. But like all precision instruments, atomic clocks are susceptible to external disturbances. This is where optical isolators come into play, ensuring that atomic clocks run with unparalleled accuracy.
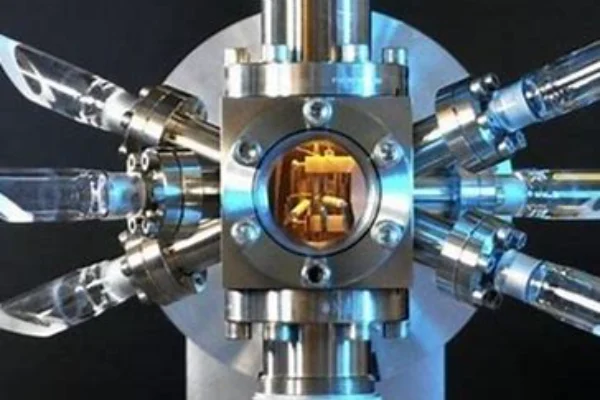
The Fundamentals of Atomic Clocks
Atomic clocks stand as marvels in the world of timekeeping, representing a significant leap from traditional methods. Unlike the clocks we often encounter in our daily lives, which depend on the intricate dance of gears or the steady rhythm of a quartz crystal, atomic clocks delve into the realm of the subatomic. Their precision and accuracy are derived from the very nature of atoms themselves.
To truly appreciate the sophistication of atomic clocks, one must first understand the fundamental principles that govern their operation. At the core of these devices lies an atom, a tiny particle that forms the building block of matter. Atoms are not static entities; they are dynamic, with electrons orbiting their nuclei in distinct energy levels or states. These energy states are not arbitrary; they are quantized, meaning electrons can only exist in specific, well-defined energy levels.
The magic of atomic clocks lies in harnessing these atomic oscillations. When an atom is subjected to a particular frequency of radiation, something fascinating happens. The electrons within the atom can absorb this energy and jump from a lower energy state to a higher one. Conversely, they can also release energy and drop to a lower energy state. This transition between energy states is predictable and consistent, making it an ideal mechanism for timekeeping.
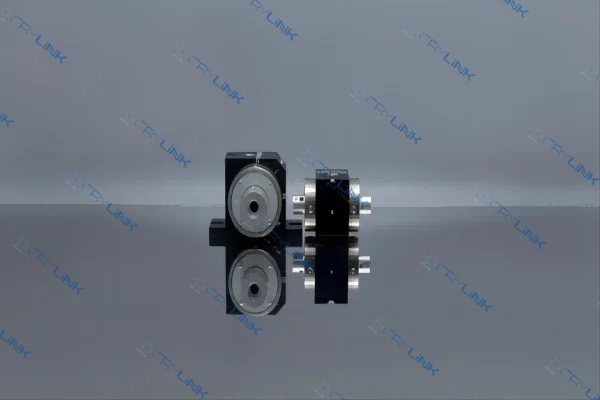
Imagine a vast, silent auditorium, with a single metronome ticking away on the stage. Each tick represents an atomic oscillation, a jump between energy states. If one were to count each tick of the metronome, they would have a precise measure of time. This is the principle behind atomic clocks. By monitoring the number of times an atom oscillates between its energy states, these clocks can measure time with an accuracy that is unparalleled by any other method.
But why atoms? The answer lies in their inherent consistency. The oscillations of atoms are governed by the fundamental laws of physics, which remain unchanged regardless of external conditions. Whether in the cold vacuum of space or the warm confines of a laboratory, the behavior of atoms remains the same. This consistency ensures that atomic clocks remain accurate, irrespective of where they are or the conditions they are subjected to.
In contrast, traditional timekeeping methods, like the ticking of a mechanical clock or the pulsing of a quartz crystal, can be influenced by various factors. Temperature changes, mechanical wear and tear, and even gravitational effects can introduce errors. Atomic clocks, with their reliance on atomic oscillations, are immune to such inconsistencies.
In conclusion, atomic clocks represent a fusion of science and engineering, bringing together quantum mechanics, electronics, and precision engineering to create devices that can measure time with astonishing accuracy. By tapping into the consistent and predictable nature of atomic oscillations, these clocks have redefined our understanding of time, setting the gold standard for precision timekeeping.
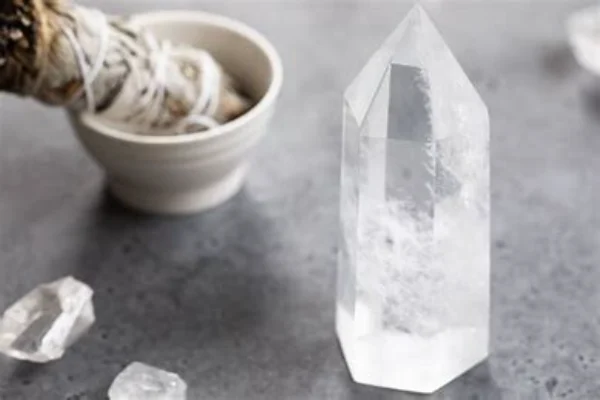
Challenges in Atomic Clock Design
Atomic clocks, despite their unparalleled precision in timekeeping, face a myriad of challenges that can potentially compromise their accuracy. These marvels of modern science, while exceptional in their design, are still susceptible to the myriad of external factors that our vast universe presents. One of the primary challenges in the design and operation of atomic clocks is the myriad of external influences that can introduce discrepancies in their readings.
Temperature fluctuations, for instance, can have a profound impact on atomic clocks. Atoms, being the fundamental units of matter, respond to changes in temperature. As temperatures rise or fall, atoms can become more or less energetic, which can, in turn, affect their oscillations. Even the slightest change in temperature can cause atoms to behave differently, leading to minute inaccuracies in time measurement.
Similarly, magnetic fields pose another challenge. Atoms, especially those with unpaired electrons, are sensitive to magnetic fields. When exposed to such fields, the atomic oscillations that these clocks rely on can be altered. Given that the Earth itself has a magnetic field, and other equipment in proximity can produce electromagnetic interference, maintaining an environment free from magnetic disturbances becomes crucial.
Then there are cosmic rays, high-energy particles from outer space that can penetrate deep into the Earth’s atmosphere. When these particles interact with an atomic clock, they can induce changes in the atomic oscillations, leading to potential inaccuracies. Given that these rays are continuously bombarding the Earth, shielding atomic clocks from their influence becomes a significant design consideration.
But beyond these specific challenges lies a broader, overarching need: stability. Atomic clocks, to function at their peak, require an environment of utmost stability. This means not just shielding them from the aforementioned external factors but also ensuring that their internal mechanisms are free from any potential sources of error. Every component, every atom, every electron within the system must be perfectly synchronized and harmonized.
In essence, the quest for perfect timekeeping is a delicate balance. While we have harnessed the power of atoms to measure time with unprecedented accuracy, ensuring that this accuracy is maintained requires a deep understanding of both the atomic world and the universe at large. It’s a testament to human ingenuity that we’ve come this far, but the journey to perfect timekeeping continues, with each challenge offering a new opportunity for discovery and innovation.
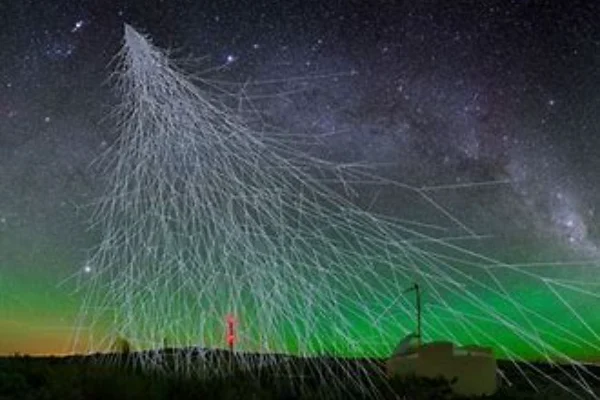
The Role of Optical Isolators in Atomic Clocks
In the intricate world of atomic clocks, optical isolators emerge as pivotal components, ensuring the purity and precision of the timekeeping process. These devices, designed to allow light to travel exclusively in one direction, serve as guardians, ensuring that the radiation interacting with the atomic oscillations remains uncontaminated. Their role is twofold. Firstly, they act as barriers, preventing any reflected or extraneous light from infiltrating the atomic clock system.
Such external light sources, if allowed to penetrate, could wreak havoc on the delicate atomic oscillations, introducing errors and inaccuracies. Secondly, optical isolators are instrumental in frequency control. By meticulously ensuring that only radiation of the correct frequency interacts with the atom, they guarantee that the atom’s oscillations remain consistent and accurate. In essence, optical isolators stand as silent sentinels, safeguarding the integrity and precision of atomic clocks against external disturbances and ensuring their unparalleled accuracy.
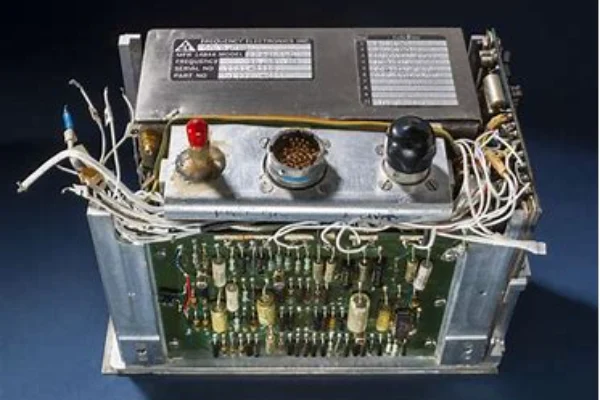
Applications of Optical Isolators in Different Atomic Clocks
Optical isolators, with their unique ability to allow light to travel in only one direction, have found significant applications in the domain of atomic clocks, enhancing their precision and reliability. Cesium atomic clocks, often regarded as the epitome of timekeeping, rely heavily on these isolators. The precision with which cesium atoms oscillate, specifically at a frequency of 9,192,631,770 times per second, is safeguarded by optical isolators. They ensure that this exact frequency, which is instrumental in defining a second, remains uncontaminated and consistent.
On the other hand, rubidium atomic clocks, though slightly less accurate than the cesium variants, bring to the table their compactness and cost-effectiveness. These clocks are more accessible to a broader audience, and the role of optical isolators in them is no less significant. By acting as a protective shield, these isolators prevent any external light, which can potentially disrupt the atomic oscillations, from interfering with the rubidium atoms, thereby ensuring the clock’s accuracy.
The world of atomic timekeeping has also seen the advent of strontium atomic clocks, a more recent innovation with the promise of even greater accuracy than their cesium counterparts. Given their nascent stage and the potential they hold, the dependency on optical isolators becomes even more pronounced. These isolators not only protect the strontium atoms from external disturbances but also play a pivotal role in the clock’s overall functionality, ensuring that the promise of enhanced accuracy is realized in practice. In essence, across various atomic clocks, optical isolators stand as silent yet crucial components, underpinning their precision and reliability.
Conclusion
Optical isolators, though unsung, play a pivotal role in the world of atomic clocks. By ensuring that these precision instruments are free from external disturbances and operate at the correct frequencies, optical isolators ensure that our understanding of time remains accurate and true.
FAQs
- What is the primary function of an optical isolator in an atomic clock?
- The primary function of an optical isolator in an atomic clock is to allow light to travel in only one direction, preventing any reflected or unwanted light from interfering with the atomic oscillations.
- How do atomic clocks differ from regular clocks?
- Unlike regular clocks that rely on mechanical movements or quartz oscillations, atomic clocks base their timekeeping on the precise oscillations of atoms.
- Why are cesium atomic clocks considered the gold standard in timekeeping?
- Cesium atomic clocks are considered the gold standard because they are the most accurate, with the second being defined by the oscillation of cesium atoms at a frequency of 9,192,631,770 times per second.
- Are strontium atomic clocks more accurate than cesium clocks?
- Strontium atomic clocks have the potential to be more accurate than cesium clocks, though research is ongoing.
- How do optical isolators enhance frequency control in atomic clocks?
- Optical isolators ensure that only the correct frequency of radiation reaches the atom, ensuring that the atom oscillates at the right frequency.
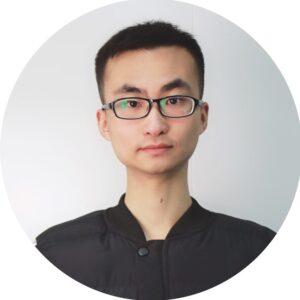
Frank
Frank graduated from the University of Shanghai for Science and Technology, majoring in optics. As a technical engineer at Crylink Company, he deeply understands crystal materials and laser components.
Related Video(s) with this Article
Related Product(s) with this Article
Related Application(s) with this Article