Chapter 1. Introduction to Laser Crystals
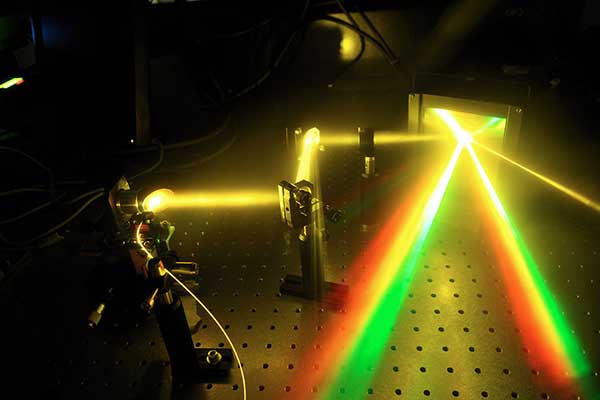
Lasers produce a beam of light that is coherent, monochromatic, and collimated. Laser crystals are the core component of lasers, providing the gain medium for the stimulated emission of photons. The efficiency, power, and wavelength of a laser depend on the properties of the laser crystal. This chapter will introduce the basic principles of laser operation and provide an overview of the different types of laser crystals and their applications.
1.1 Principles of Laser Operation
The word “laser” is an acronym for “Light Amplification by Stimulated Emission of Radiation.” Laser operation is based on population inversion, stimulated emission, and amplification principles. A population inversion occurs when more atoms or molecules are in an excited state than in the ground state. Stimulated emission occurs when a photon stimulates an excited atom or molecule to release a second photon that is coherent with the first one. Amplification occurs when photons are repeatedly stimulated and emitted in phase with each other, resulting in a high-intensity beam of coherent light.
The process of laser operation can be summarized in four steps:
- Pumping: Energy is input into the laser crystal, usually in the form of light or electricity, to create a population inversion.
- Absorption: The excited atoms or molecules absorb photons of a specific wavelength, raising them to a higher energy level.
- Stimulated Emission: When a photon of the same wavelength as the absorbed photon strikes an excited atom or molecule, it stimulates the emission of a new photon.
- Amplification: The new photon is emitted in phase with the stimulating photon, which amplifies the light.
1.2 Types of Laser Crystals
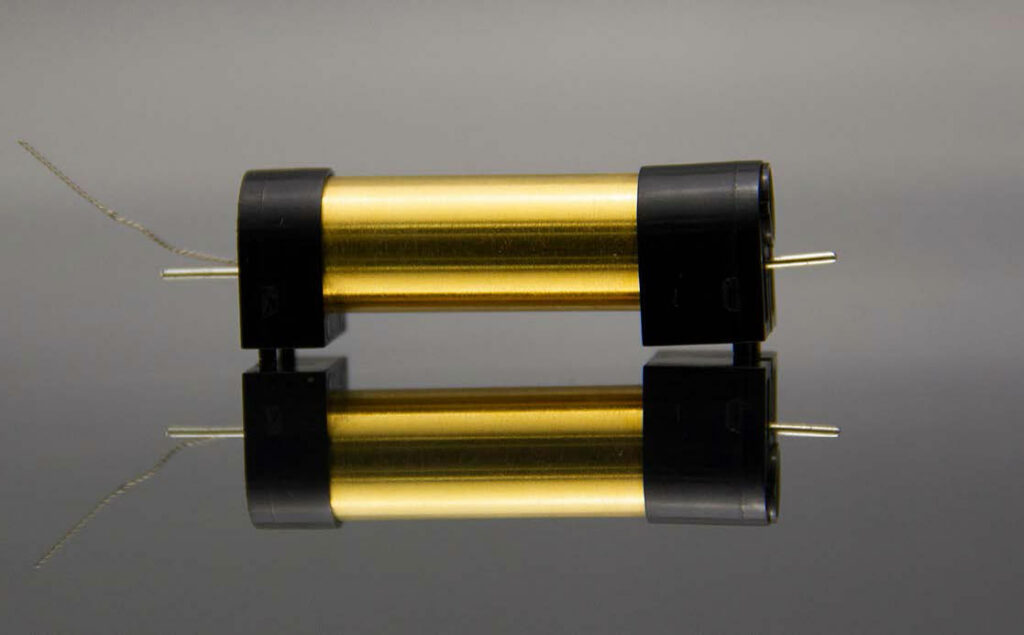
There are various types of laser crystals, including solid-state, gas, and semiconductor lasers. Solid-state lasers are the most common type of laser and consist of a laser crystal as the gain medium. The laser crystal is usually doped with ions of a transition metal, rare earth element, or semiconductor material.
Some of the most common laser crystals used in solid-state lasers include:
- Ti Sapphire Crystal: Ti Sapphire Crystal is a widely used laser crystal due to its broad tuning range, high gain, and excellent thermal and mechanical properties. Ti Sapphire Crystal lasers are used in various applications, including materials processing, medical research, and spectroscopy.
- Nd: YAG: Nd: YAG is one of the most widely used laser crystals due to its high efficiency, low threshold, and excellent thermal properties. Nd: YAG lasers are used in various applications, including laser cutting, welding, and marking.
- Nd: YVO4: Nd: YVO4 is a laser crystal that has become increasingly popular due to its high gain, high efficiency, and excellent thermal properties. Nd: YVO4 lasers are used in various applications, including laser micromachining, material processing, and medical research.
- Yb:KGW [Yb: KGd(WO4)2]: Yb: KGd(WO4)2 is a laser crystal that has recently gained popularity due to its high gain, high efficiency, and broad wavelength coverage. Yb: KGd(WO4)2 lasers are used in various applications, including laser cutting, welding, and marking.
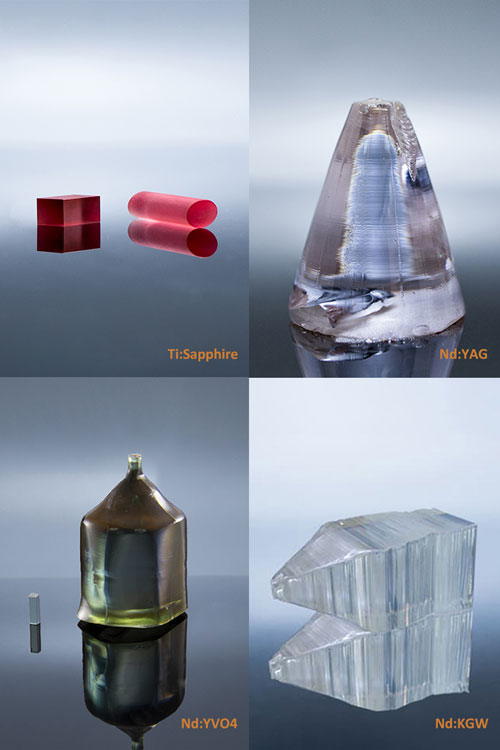
1.3 Applications of Laser Crystals
Laser crystals have a wide range of applications in various fields, including medicine, communication, manufacturing, and research. Some of the most common applications of laser crystals include:
- Medical Applications: Laser crystals are used in medical applications such as laser surgery, cosmetic procedures, and ophthalmology.
- Communication: Laser crystals are used in fiber-optic communication to transmit information over long distances with high speeds and low signal loss.
- Manufacturing: Laser crystals are used in manufacturing processes such as cutting, welding, and marking.
- Research: Laser crystals are used in research applications such as spectroscopy, microscopy, and optical sensing.
The choice of laser crystal depends on the specific application requirements, such as the desired output wavelength, power, and efficiency.
1.4 Conclusion
In this chapter, we have introduced the basic principles of laser operation and provided an overview of the different types of laser crystals and their applications. Laser crystal play a critical role in determining a laser’s efficiency, power, and wavelength. Ti Sapphire Crystal is a widely used laser crystal due to its broad tuning range, high gain, and excellent thermal and mechanical properties. Other laser crystals, such as Nd: YAG, Nd: YVO4, and Yb: KGd(WO4)2, also offer unique properties that make them suitable for specific applications. In the following chapters, we will detail the structure, optical and thermal properties, laser operation, frequency conversion, and applications of these laser crystals.
Chapter 2. Crystal Structure and Growth
The crystal structure and growth of laser crystals have a significant impact on their optical and thermal properties, as well as their laser performance. This chapter will discuss the different crystal structures of laser crystals and their impact on laser performance. We will also explain the different growth techniques used to produce high-quality laser crystals and their advantages and disadvantages.
2.1 Crystal Structures of Laser Crystals
Laser crystals have different crystal structures, including cubic, tetragonal, orthorhombic, and monoclinic. The crystal structure determines the symmetry and orientation of the crystal lattice, as well as the optical and thermal properties of the crystal. Some of the most common crystal structures of laser crystals are discussed below.
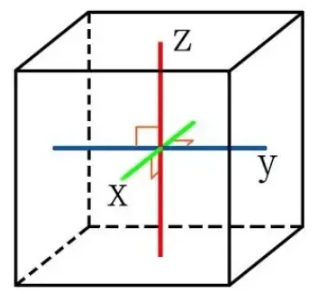
Cubic: The cubic crystal structure has a high degree of symmetry and is characterized by equal lengths of all sides and angles of 90 degrees. Nd: YAG and Yb: YAG are examples of laser crystals with a cubic structure. The cubic structure of Nd: YAG results in high thermal conductivity, making it an ideal laser crystal for high-power applications.
Tetragonal: The tetragonal crystal structure is characterized by equal lengths of two sides and angles of 90 degrees. Ti Sapphire Crystal has a tetragonal structure, which results in a broad tuning range and high gain. The tetragonal structure also provides high mechanical stability, making Ti Sapphire Crystal suitable for high-power applications.
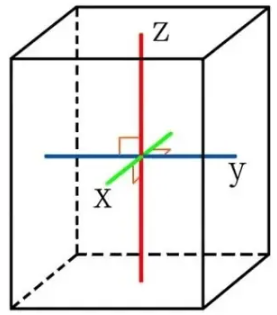
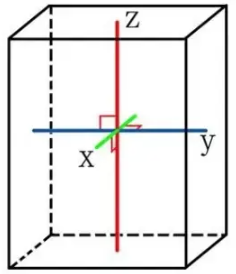
Orthorhombic: The orthorhombic crystal structure has three unequal sides and angles of 90 degrees. Nd: YVO4 is an example of a laser crystal with an orthorhombic structure. The orthorhombic structure of Nd: YVO4 results in high gain and efficiency, making it an ideal laser crystal for high-power applications.
Monoclinic: The monoclinic crystal structure has unequal sides and angles that are not 90 degrees. This crystal structure is found in laser crystals, such as Cr: YAG and Er: YAG. The monoclinic structure of Cr: YAG results in a broad absorption band, making it suitable for tunable laser applications.
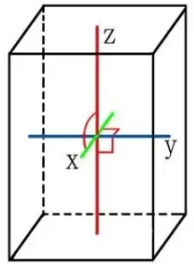
2.2 Crystal Growth Techniques
Crystal growth creates a large single crystal from a small seed crystal. The quality of the crystal depends on the growth technique used. Several crystal growth techniques are used to produce high-quality laser crystals, including Czochralski, Bridgman, and floating zone methods.
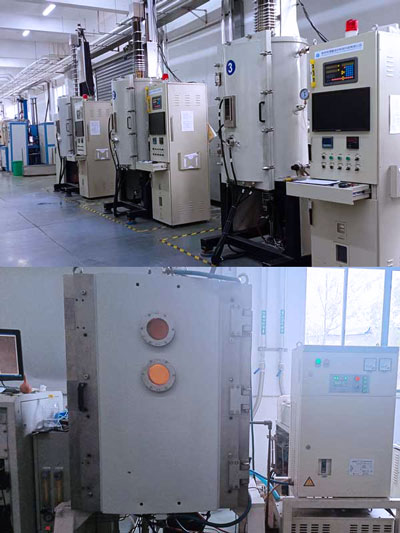
- Czochralski Method: The Czochralski method is the most common crystal growth technique for producing laser crystals. This technique involves melting a small amount of crystal material in a crucible and pulling a single crystal from the melt. The crystal is slowly rotated and pulled out of the melt to maintain a constant temperature gradient. The Czochralski method is widely used because it produces large, high-quality crystals with minimal defects.
- Bridgman Method: Bridgman involves melting a small amount of crystal material in a crucible and slowly cooling the melt from one end to produce a single crystal. The crystal is grown by moving the crucible and cooling zone through the melt at a constant speed. The Bridgman method is used to produce high-quality laser crystals with low dislocation densities.
- Floating Zone Method: The floating zone method involves melting a small amount of crystal material by focusing a high-intensity light beam on a small area of the crystal. The crystal is slowly moved through the melt to produce a single crystal. The floating zone method produces high-quality laser crystals with minimal defects and high purity.
2.3 Crystal Defects
Crystal defects, such as dislocations, point defects, and impurities, can significantly impact the optical and thermal properties of laser crystals. Dislocations are crystal lattice structure defects resulting from crystal growth processes or mechanical stresses. Point defects occur when an atom or molecule is missing from or added to the crystal lattice. Impurities are foreign atoms or molecules that are incorporated into the crystal lattice during crystal growth.
Crystal defects can cause a reduction in crystal quality, resulting in reduced optical and thermal properties. Dislocations can cause light scattering and thermal stress, leading to cracking and reduced laser performance. Point defects can cause optical losses and reduce laser efficiency. Impurities can cause absorption and emission at undesired wavelengths, resulting in reduced laser performance.
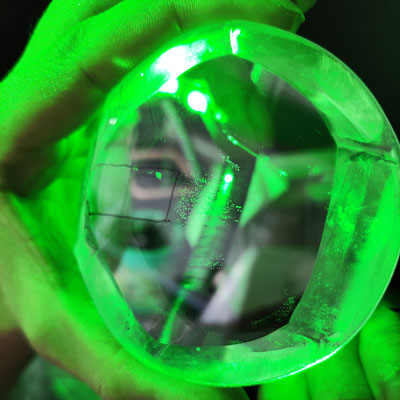
Various techniques are used during crystal growth to reduce the impact of crystal defects, such as annealing, doping, and polishing. Annealing is heating the crystal to a high temperature to remove defects and improve crystal quality. Doping introduces impurities into the crystal lattice to modify the crystal properties, such as the refractive index or gain. Polishing is the process of smoothing the crystal surface to reduce scattering and improve laser performance.
2.4 Conclusion
This chapter discusses the crystal structure and growth of laser crystals and their impact on optical and thermal properties and laser performance. Laser crystals have different crystal structures, including cubic, tetragonal, orthorhombic, and monoclinic, which determine their properties and applications. Crystal growth techniques, such as Czochralski, Bridgman, and floating zone methods, produce high-quality laser crystals with minimal defects.
Crystal defects, such as dislocations, point defects, and impurities, can significantly impact laser performance and are minimized using various techniques, such as annealing, doping, and polishing. In the following chapters, we will delve into the optical and thermal properties, laser operation, frequency conversion, and applications of laser crystals in more detail.
Chapter 3. Optical and Thermal Properties of Laser Crystals
Laser crystals’ optical and thermal properties play a critical role in determining their laser performance. This chapter will discuss the optical and thermal properties of laser crystals, including absorption, emission, fluorescence lifetime, refractive index, and thermal conductivity. We will also compare the properties of Ti Sapphire Crystal and other laser crystals.
3.1 Optical Properties of Laser Crystals
The optical properties of laser crystals include absorption, emission, fluorescence lifetime, and refractive index. These properties determine the laser performance of the crystal, including the output wavelength, gain, and efficiency.
- Absorption: Absorption is the process by which the crystal absorbs photons of a specific wavelength. The absorption spectrum of a laser crystal depends on its doping concentration and crystal structure. For example, Ti Sapphire Crystal has a broad absorption spectrum extending from ultraviolet to near-infrared.
- Emission: Emission is the process by which photons are emitted from the crystal. The emission spectrum of a laser crystal depends on its doping concentration and crystal structure. For example, Nd: YAG emits at a wavelength of 1.06 microns, while Ti Sapphire Crystal emits in the visible and near-infrared regions.
- Fluorescence Lifetime: Fluorescence lifetime is the time it takes for a fluorophore to return to its ground state after being excited. The fluorescence lifetime of a laser crystal depends on its doping concentration and crystal structure. For example, Ti Sapphire Crystal has a long fluorescence lifetime of several microseconds, while Nd: YAG has a short fluorescence lifetime of a few nanoseconds.
- Refractive Index: Refractive index is the ratio of the speed of light in a vacuum to the speed of light in the crystal. A laser crystal’s refractive index depends on its structure and composition. For example, Ti Sapphire Crystal has a high refractive index of 1.76, while Nd: YAG has a low refractive index of 1.82.
3.2 Thermal Properties of Laser Crystals
The thermal properties of laser crystals include thermal conductivity, thermal expansion, and specific heat capacity. These properties determine the ability of the crystal to dissipate heat and maintain its structural integrity during laser operation.
- Thermal Conductivity: Thermal conductivity is the ability of a material to conduct heat. The thermal conductivity of a laser crystal depends on its crystal structure and composition. For example, Nd: YAG has a high thermal conductivity of 14 W/mK, while Ti Sapphire Crystal has a lower thermal conductivity of 9 W/mK.
- Thermal Expansion: Thermal expansion is the tendency of a material to expand when heated. The thermal expansion coefficient of a laser crystal depends on its crystal structure and composition. For example, Ti Sapphire Crystal has a low thermal expansion coefficient of 5.8×10^-6 K^-1, while Nd: YAG has a higher thermal expansion coefficient of 7.8×10^-6 K^-1.
- Specific Heat Capacity: Specific heat capacity is the amount of heat required to raise the temperature of a material by one degree Celsius. The specific heat capacity of a laser crystal depends on its crystal structure and composition. For example, Ti Sapphire Crystal has a low specific heat capacity of 0.59 J/gK, while Nd: YAG has a higher specific heat capacity of 0.67 J/gK.
3.3 Comparison of Ti Sapphire Crystal and Other Laser Crystals
Ti Sapphire Crystal has unique optical and thermal properties, making it a popular laser crystal for various applications. Ti Sapphire Crystal has a broad tuning range, high gain, and excellent thermal and mechanical properties compared to other laser crystals. YAG has high thermal conductivity and is commonly used for high-power laser applications. Nd: YVO4 has high gain and efficiency, making it suitable for high-power laser applications. Yb: KGW has a high absorption cross-section and is used for high-power laser applications, frequency doubling, and parametric oscillation.
When choosing a laser crystal for a specific application, it is important to consider the desired output wavelength, power, efficiency, and the crystal’s optical and thermal properties. Ti Sapphire Crystal is a versatile laser crystal that can be used for a wide range of applications due to its broad tuning range and high gain. However, Nd: YAG, Nd: YVO4, and Yb: KGd(WO4)2 are often preferred for high-power applications due to their high thermal conductivity and efficiency.
3.4 Conclusion
In this chapter, we have discussed laser crystals’ optical and thermal properties and their impact on laser performance. The optical properties of laser crystals, such as absorption, emission, fluorescence lifetime, and refractive index, determine the laser performance of the crystal. The thermal properties of laser crystals, such as thermal conductivity, thermal expansion, and specific heat capacity, determine the ability of the crystal to dissipate heat and maintain its structural integrity during laser operation.
Ti Sapphire Crystal has unique optical and thermal properties, making it a popular laser crystal for various applications. When choosing a laser crystal for a specific application, it is important to consider the desired output wavelength, power, efficiency, and the crystal’s optical and thermal properties. In the following chapters, we will detail the laser operation, frequency conversion, and applications of laser crystals.
Chapter 4. Laser Operation of Laser Crystals
The laser operation of laser crystals is a complex process involving light absorption, amplification, and emission. This chapter will discuss the different laser operation modes of laser crystals, including continuous wave (CW), pulsed, and mode-locked operation. We will also explain the factors that affect laser performance, such as the pumping scheme, crystal length, and cavity design.
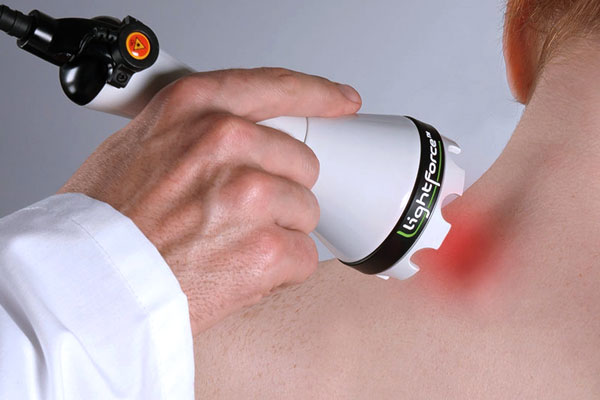
4.1 Continuous Wave Operation
Continuous wave (CW) operation is a laser operation mode in which the laser emits a continuous wave of light. In CW operation, the laser is continuously pumped to maintain a population inversion in the laser crystal. The output power of a CW laser is proportional to the pump power and the efficiency of the laser crystal.
CW lasers are commonly used in material processing, spectroscopy, and medical applications. Ti Sapphire Crystal is a popular laser crystal for CW operation due to its broad tuning range and high gain.
4.2 Pulsed Operation
Pulsed operation is a laser operation mode in which the laser emits short light pulses. In pulsed operation, the laser is pumped with a high-energy pulse to create a population inversion in the laser crystal. The output power of a pulsed laser is proportional to the pulse energy and repetition rate.
Pulsed lasers are commonly used in applications such as laser ablation, micromachining, and laser-induced breakdown spectroscopy. Nd: YAG is a popular laser crystal for pulsed operation due to its high efficiency and short fluorescence lifetime.
4.3 Mode-Locked Operation
Mode-locked operation is a laser operation mode in which the laser emits a train of ultra-short pulses of light. In mode-locked operation, the laser cavity is designed to create a series of resonant modes that interact with each other to produce a train of ultra-short pulses. The output power of a mode-locked laser is proportional to the pump power and the efficiency of the laser crystal.
Mode-locked lasers are commonly used in applications such as femtosecond spectroscopy, optical coherence tomography, and multiphoton microscopy. Ti Sapphire Crystal is a popular laser crystal for mode-locked operation due to its broad tuning range and high gain.
4.4 Factors Affecting Laser Performance
Several factors affect the laser performance of laser crystals, including the pumping scheme, crystal length, and cavity design.
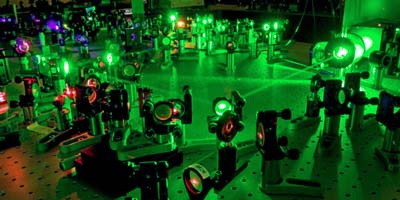
Pumping Scheme: The pumping scheme determines the method used to excite the laser crystal and create a population inversion. The most common pumping schemes are optical pumping and electrical pumping. Optical pumping involves using a flash lamp or diode laser to excite the laser crystal, while electrical pumping involves using a high-voltage discharge to excite the laser crystal.
Crystal Length: The crystal length determines the amount of gain and the saturation energy of the laser crystal. A longer crystal length results in a higher gain and higher saturation energy, while a shorter crystal length results in a lower gain and lower saturation energy.
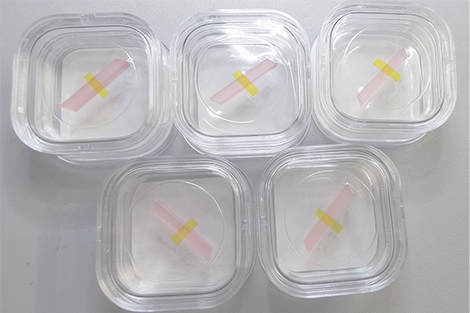
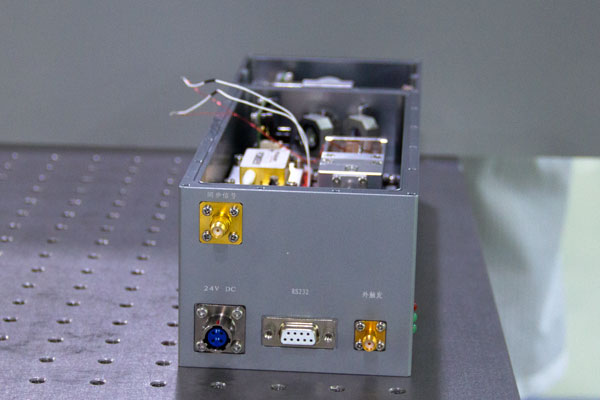
Cavity Design: The cavity design determines the laser’s mode structure and output power. The most common cavity designs are linear cavities and ring cavities. Linear cavities provide high output power and single-mode operation, while ring cavities provide low output power and multi-mode operation.
4.5 Conclusion
In this chapter, we have discussed the laser operation of laser crystals and the different laser operation modes, including continuous wave, pulsed, and mode-locked operation. We have also explained the factors that affect laser performance, such as the pumping scheme, crystal length, and cavity design. Laser crystals play a critical role in determining laser performance. The choice of laser crystal depends on the specific application requirements, such as the desired output power, wavelength, and efficiency.
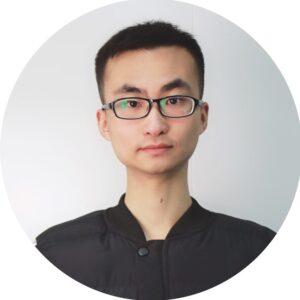
Frank
Frank graduated from the University of Shanghai for Science and Technology, majoring in optics. As a technical engineer at Crylink Company, he deeply understands crystal materials and laser components.
Related Video(s) with this Article
Related Product(s) with this Article
Related Application(s) with this Article