What are the prospects for high-energy solid-state lasers?
Since the advent of laser technology in 1960, solid-state laser technology has made tremendous advancements, leading the way in peak power among various types of lasers, reaching 1014 watts. Particularly in the field of laser nuclear fusion, significant breakthroughs have been achieved by utilizing the high peak power of solid-state lasers. Entering the 21st century, especially with the support of the U.S. military’s Joint High Power Solid-State Laser (JHPSSL) and Robust Electric Laser Initiative (RELI) programs, new laser technologies employing high-power semiconductor pumping (such as slab lasers, disk lasers, and fiber lasers) have seen significant improvements in output power and efficiency. With the industrial demand for advanced laser manufacturing technologies, semiconductor-pumped solid-state lasers have entered a golden age of development. High-energy solid-state lasers play a vital role in various fields such as industry, medicine, military, and scientific research.
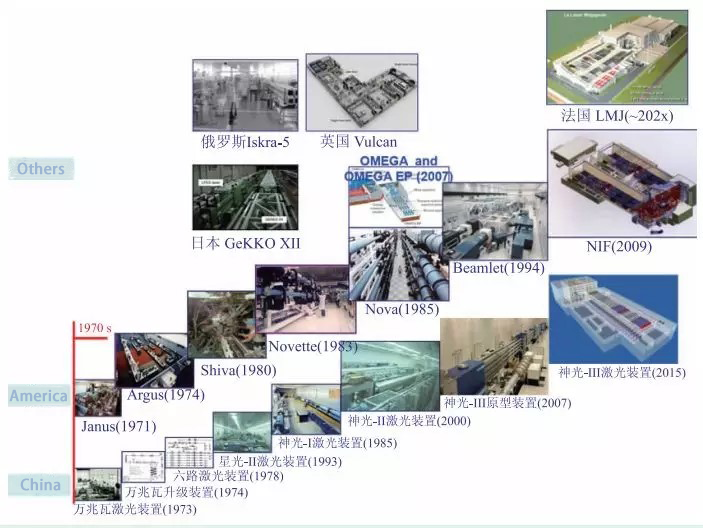
Figure 1. Development history of high energy solid laser equipment
Industrial
In the field of industrial manufacturing, high energy solid state lasers can be used for cutting, welding, material processing, etc. Their high precision and high efficiency make the production process more efficient and economical, especially suitable for high-tech fields requiring precision machining.
Medical
In the medical field, high energy solid lasers can be used in eye surgery, tumor treatment, skin disease treatment, etc. They offer a non-invasive form of treatment that can reduce surgical risks and improve treatment outcomes.
Military Science
In the military, high-energy solid-state lasers are used for precision strikes, air and sea defense systems, and reconnaissance missions. They provide a high-precision, fast-response means of warfare that can effectively enhance defense capabilities and improve strategic flexibility on the battlefield. This laser technology enables the military to quickly and accurately locate and neutralize threats in different environments, such as on the ground, in the air and at sea. In addition, high energy lasers also play a key role in target identification, distance measurement, and interference and destruction of enemy electronic devices, becoming an indispensable high-tech weapon in modern warfare.
Scientific Research
High energy solid state laser also plays an important role in physics, chemistry and other scientific research. They can be used to study the fundamental properties of matter, such as to explore new forms of energy through laser-induced nuclear fusion experiments.
What are the problems encountered in the development of high energy solid state lasers?
The development of high average power solid-state laser technology has always been centered around two core themes: one is to improve the output power of the laser, and the other is to improve the quality factor of the laser beam. However, these two goals are often mutually restrictive. Specifically, under the operating conditions of high power pumping, the waste heat generated by the laser gain medium will radiate from its surface, forming a temperature gradient. This uneven temperature distribution leads to thermo-optical distortion, which deteriorates the beam quality of the output laser. In addition, the thermal load the gain material itself is subjected to is also a key determinant of its thermal stress limit. This thermal stress limit directly affects the size of the material and the energy storage capacity, which in turn limits the maximum output power of the laser.
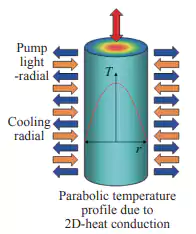
Figure 2. Solid laser configuration — rod laser gain medium
Diode-pumped all-solid-state lasers are favored for their high efficiency, good beam quality, stable working performance, small size, low cost and easy maintenance. This kind of laser has been widely used in many fields such as laser processing, scientific research and national defense. However, the radial temperature gradient produced by the traditional rod-like laser gain medium during cooling brings about a series of thermal effects, such as thermal lensing phenomenon and stress depolarization. These thermal effects ultimately lead to a reduction in the output power of the laser and a reduction in the beam quality, thus limiting the possibility of the development of this class of lasers to higher average power.
Thermal Lens Effect
The total thermal lens effect of crystal includes three parts: temperature gradient, stress birefringence and end deformation. When the laser crystal is focused by the pump light during high-power density pumping, high energy density is generated inside the crystal, resulting in the crystal end deformation becoming more obvious, which accounts for an increasingly important proportion of the total thermal lensing effect.
- The thermal lens effect caused by the temperature gradient originates from the spatially uneven radial temperature gradient distribution generated by the gain medium absorbing the pump light energy. This non-uniformity of temperature distribution results in the spatial distribution of axial thermal stress. Due to the combined effect of the radial temperature gradient and axial thermal stress inside the crystal, the refractive index within the laser crystal changes with temperature, optically appearing as a lens with a gradient refractive index.
- The thermal lensing effect caused by thermal stress birefringence is the thermal stress caused by the non-uniform distribution of temperature in the gain crystal. The thermal stress changes the refractive index through photoelastic effect, which makes the original isotropic crystal material become anisotropic, resulting in birefringence. Or to change the original birefringence properties of materials that already have anisotropy. This is the thermal stress birefringence effect.
- The thermal lens effect caused by end-face deformation is due to the accumulation and non-uniform distribution of temperature in the laser crystal caused by end-pumped gain medium. Due to the inherent thermal expansion and cold contraction of solid materials, the crystal produces non-directional deformation. At the same time, due to the continuous arrangement of crystal lattice and heat conduction, the crystal end face will produce nonlinear expansion deformation, resulting in the formation of thermal lensing effect.
What is the solution to the thermal effects?
In order to solve the thermal effect in high energy solid-state lasers, researchers have proposed a variety of new laser medium configurations, including slatted, wafer and fiber lasers. In particular, the slatted laser adopts a zigzag optical path to transmit the laser, which effectively eliminates the first-order thermal focusing and thermal birefringence effects. This design significantly improves the uniformity of the beam wavefront, and achieves better beam quality and higher output power than conventional rod-like lasers.
The zigzag lath configuration is shown in the figure below. In this configuration, the laser beam travels inside the slats via total internal reflection and extracts energy in a zigzag path. This configuration can be seen as the result of multiple activation mirrors connected in series. It not only provides efficient energy extraction and compact structural design, but also allows two large surfaces to serve as windows for pumping and heat dissipation at the same time, further enhancing the performance of the laser. Performance and efficiency. Through such a design, the slab laser has significant advantages in handling thermal effects, providing a new direction for the development of solid-state laser technology.
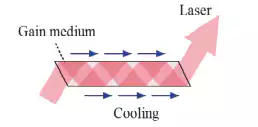
Figure 3. Zigzag slats
Development history of slab laser
Since Martin proposed the slat configuration in 1972, research work in this field has made significant progress. Researchers have used a variety of pumping technologies, including side pumping, end pumping and angle pumping of diode lasers, to achieve major technological breakthroughs.
In 2000, Hagop Injeyan and others proposed a CCE (Conductioncooledend-pumpedslab) laser solution based on the principle of slab lasers and combined with conduction cooling and end-pumping technology. Then in 2001, Stephen Palese and others used CCEPS (Conduction Cooled End-Pumped Slab) as an amplifier and successfully obtained a 150W linearly polarized output with a beam quality M2 value of 1.25. In 2005, Northrop Grumman developed the The VESTA system consists of four CCEPS amplifiers with an output power of up to 5kW. The beam quality reaches 1.3 times the diffraction limit, and the beam quality does not decrease significantly after 20 minutes of stable operation.
With the continuous development and improvement of slat laser technology, especially in the gradual solution of key problems such as high brightness pump coupling, efficient heat dissipation and end deformation, slat laser has achieved a 100kW output power for the first time, and has gradually become a representative solution of high average power solid laser technology. These achievements mark the important position of slatted laser technology in the field of solid-state lasers, and lay a solid foundation for the research and development of higher power and higher efficiency solid-state lasers in the future.
Magnified overview of slats
The full name of the slab amplifier is “slat geometry laser” (SGL for short), which is a special solid-state laser. It has a slat-like shape and the laser travels along the length of the medium during operation. The power level of this amplifier is the highest, and the only two solid-state laser systems in the world with power exceeding 100 kilowatts both use slat structures.
Its advantages are: small size, simple structure, increase the heat dissipation area, reduce the temperature of the laser medium, change the direction of the heat flow, change the impact of the laser through the working substance, so that it is possible to obtain high energy, high power, high beam quality laser output. Although the shape of the laser medium is changed, the excitation transition characteristics of the laser medium itself are not changed.
The gain media used in slab amplification lasers mainly include the following:
- Neodymium-doped crystals: such as neodymium-doped yttrium aluminum garnet (Nd:YAG). This is one of the most common gain media and is widely used due to its good thermal properties and efficient laser output.
- Yb (Ytterbium) doped material: such as ytterbium-doped glass or crystal (e.g. Yb:YAG). Ytterbium-doped materials are particularly popular in high-power applications because they have a lower thermal load and a wider absorption bandwidth.
- Erbium-doped or scandium-doped glass: These materials are used for laser output at certain wavelengths and have unique spectral properties.
- Other rare earth element doped crystals: such as holmium (Ho), thulium (Tm) and erbium (Er) doped crystals, these are often used in specific wavelength applications.
Each gain medium has its own unique characteristics, such as absorption and emission wavelengths, thermal conductivity, and upconversion efficiency, which determine its suitability for different types of slab amplified lasers. When designing a slab-amplified laser, the appropriate gain medium is selected based on factors such as required output power, wavelength, beam quality, and system efficiency.
Slat amplification research process
Since the early development of high average power in solid-state laser technology, it has been focused on the two main directions of increasing power and improving beam quality, but there is a mutually restrictive relationship between the two. Under high-power pump loads, a large amount of waste heat will be emitted from the surface of the gain medium, resulting in temperature gradients and thermo-optical distortion, which will reduce the beam quality of the output laser.
In the early development stage of slab laser, due to the limitation of flash lamp brightness, double large-surface pumping was mainly used to achieve high-power pumping (Figure 4). However, there are several problems with this method: (1) Due to insufficient pump intensity, only Nd:YAG can be selected as the gain medium at room temperature; (2) In order to transmit the pump light, the two cooling surfaces of the slats can only be used Water cooling, which limits the cooling capacity; (3) the slab must be highly doped in order to effectively absorb the pump light, which may lead to severe concentration quenching effects for Nd:YAG slabs.
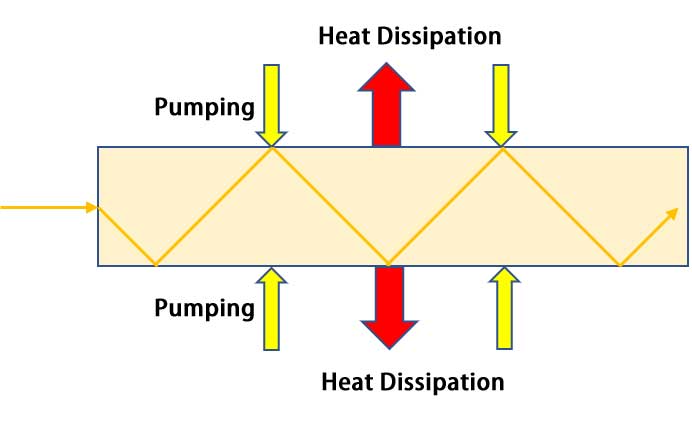
Figure 4. Schematic diagram of continuous wave pumping lath laser technology
By 1972, Martin and Chernock first proposed to rely on the laser to take a “zigzag” optical path within the slab to solve the problem of intrinsic optical distortion caused by large-area cooling of the slab. The traditional round rod-shaped laser gain medium produces a radial temperature gradient during the cooling process (Figure 5), which causes thermal effects such as thermal lensing and stress depolarization, ultimately leading to a decrease in output power and reduced beam quality. In order to reduce the influence of thermal distortion in solid-state lasers, thin-sheet lasers (Figure 6) and zigzag optical path slab lasers (Figure 7) have been developed. The basic idea of the latter two is that during the laser extraction process, a certain directional component of the laser propagation direction coincides with the direction of the temperature gradient; in this way, the beam wavefront will not be affected by the temperature gradient under the condition of first-order approximation. In 1989, Kuchar suggested welding the slab to the metal heat sink for conduction cooling, but this caused the end surface of the slab to deform due to the absorption of pump light, affecting the beam quality.
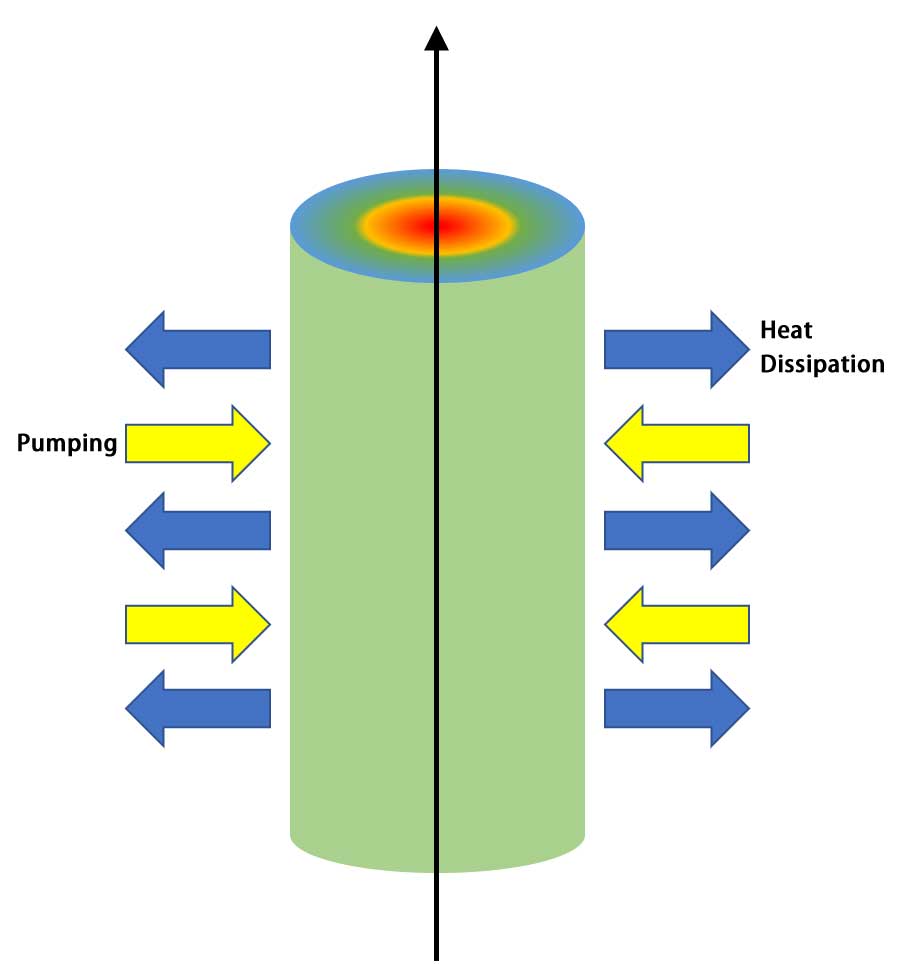
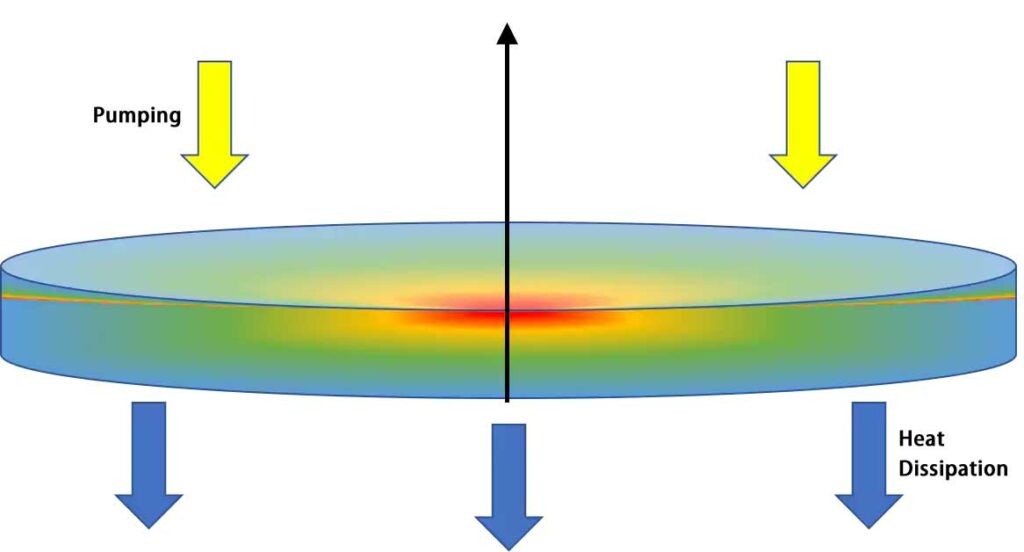
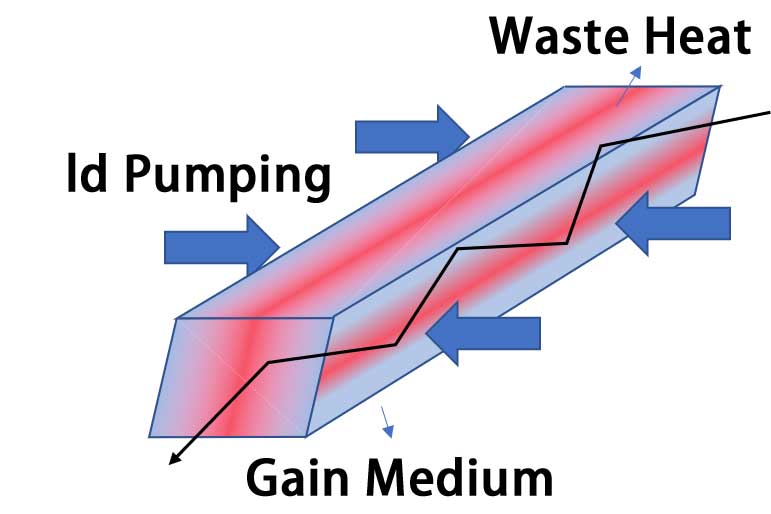
Picture 5
Picture 6
Picture 7
Figure 567. Improvement of thermo-optical distortion by changing solid laser configuration
This problem was solved in 2000 by bonding undoped YAG crystals at both ends of the slats. Gruman used a master oscillator + amplifier (MOPA) structure in 2005 to achieve a 12.5kW laser output in its amplifier link through Angle gating technology, effectively balancing the laser flux between the amplifier modules and achieving approximate energy extraction. In 2007, the output power was further increased to 15.3kW, the electro-optic conversion efficiency was 19.3%, the beam quality reached 1.58 times the diffraction limit after the active control of adaptive optics, and finally in 2009 through the coherent synthesis of 7 MOPA links to achieve 105kW laser output.
In addition to surface pumping and end-pumping solutions, slab lasers have also tried edge pumping solutions in their development history. However, the temperature gradient caused by the exponential absorption of the pump light along the width direction of the slab brings optical distortion that is difficult to solve. . The thermal lens effect it causes becomes more and more serious as the amplification optical path of the laser increases, so modern high-average power slab lasers basically do not use edge pumping schemes.
In short, in order to increase the average power of the laser, researchers initially used flash lamp pumping and later developed double large-area pumping. However, they faced multiple limitations, such as pump brightness, cooling efficiency, and doping concentration. Solutions such as low-doping slabs, conduction cooling of metal heat sinks, end-face pumping, and end-face bonding of undoped YAG crystals have gradually improved pump efficiency and beam quality. In 2005, an important breakthrough of 12.5kW output was achieved, which was further improved to 105kW through MOPA structure and coherent synthesis technology.
What are the lath medium?
Solid state laser gain media are mainly divided into two categories: glass and crystal. Of the two, a significant advantage of the glass matrix material is that it can be manufactured into very large volumes. This feature is particularly important in high-power laser operations, as it is able to provide a more powerful energy output. In contrast, glass matrix materials also show several other advantages in laser material applications: its absorption line range is wider than crystal matrix, providing a more flexible laser wavelength selection; In addition, glass substrates are generally superior to crystals in terms of doping uniformity and optical quality.
On the other hand, using crystals as a substrate for working substances also has its own unique advantages. Crystalline materials generally have a higher hardness, which makes them more reliable in terms of physical strength. The crystal’s fluorescence spectral lines are narrower, which is beneficial to producing more precise and clear laser output. Also, the crystals have higher thermal conductivities, which means they can manage heat more efficiently during high-power operation, reducing heat-induced performance degradation.
In general, the choice of glass or crystal as the laser gain medium depends on the specific application requirements and laser design. Each material has unique properties and advantages that play an important role in different laser systems.
Laser Crystal
The activation of laser crystals mainly depends on the type of ions doped in it. These ions are generally divided into transition metal ions (such as chromium (Cr3+), titanium (Ti3+), nickel (Ni2+), cobalt (Co2+)), rare earth ions (including Neodymium (Nd3+), Erbium (Er3+), Holmium (Ho3+), Praseodymium (Pr3+), Gadolinium (Gd3+), Europium (Eu3+), Ytterbium (Yb3+), Samarium (Sm2+), Dysprosium (Dy2+), Thulium (Tm2+) and actinide ions (such as uranium (U3+). Of the more than 300 types of laser crystals discovered so far, more than 90% are doped with rare earth elements as activated ions. From the perspective of practical applications, the most commonly used include neodymium-doped yttrium aluminum garnet (Nd:YAG), neodymium-doped yttrium aluminate (Nd:YVO4) and titanium-doped sapphire (Ti:Al2O3). These three are widely considered They are the “three cornerstones of laser crystals”.
Each crystal is suitable for different applications due to its unique physical and optical properties. For example, Nd:YAG crystals are often chosen as the material of choice for high-power lasers due to their ability to deliver high power. In the design of low-power and miniaturized lasers, Nd:YVO4 is favored because of its high efficiency and smaller size. For applications that require ultra-fast and tunable light sources, such as certain scientific research and medical fields, Ti:Al2O3 crystals are ideal.
1.Nd:YAG
Nd:YAG (neodymium-doped yttrium aluminum garnet) is not only one of the earliest discovered and most well-known laser crystals, but also the most widely used laser material. Whether in the small, medium or large power range, or in specialized applications such as Q-switched and mode-locked ultra-short pulse lasers, Nd:YAG is the laser working material of choice. It can be used in diode-pumped all-solid-state micro-miniature lasers to obtain high-quality continuous laser output of red, green, and blue. In addition, Nd:YAG is also widely used in military, scientific research, medical and industrial fields, including various specifications of rangefinders, photoelectric countermeasures equipment systems, high-performance laser instruments, laser treatment equipment, beauty instruments, laser marking machines and marking machines. Hole machines and other laser processing equipment.
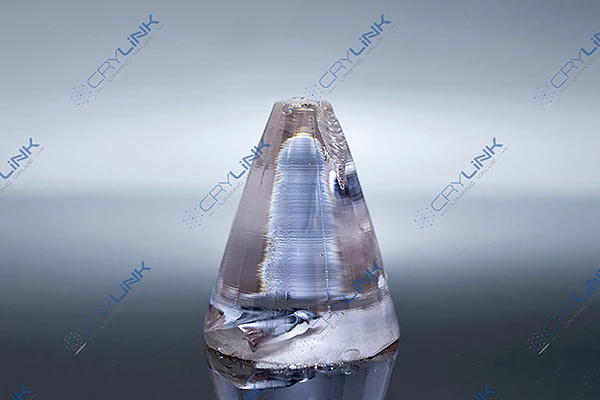
Figure 8. Nd:YAG crystal
Example
1. Duke Ming and others first proposed the Innoslab mixed-cavity slab laser at the end of the last century. The device slab crystal is made of Nd:YAG material, with a doping concentration of 1at.% and a size of 16mm*8mm*3mm.
2. In 2001, T.S.Rutherford, W.M.Tulloch and others from Stanford University used Nd:YAG as the working medium and LD side-pumped slab laser. The doping concentration of the Nd:YAG crystal is 1at%, and the size is 1.45mm×4.75mm×35.7mm.
3. In 2023, Zhang Xinghu et al. reported a high-power quasi-continuous operation all-solid-state Nd:YAG laser. The Nd∶YAG lath crystal with a trapezoidal cross-section and a doping concentration of 0.9% is welded on the microchannel cooling heat sink through the lower surface. The size of the lower large surface of the lath crystal is 96mm×30mm, the size of the upper large surface is 92mm×30mm, and the thickness of the lath is 3mm.
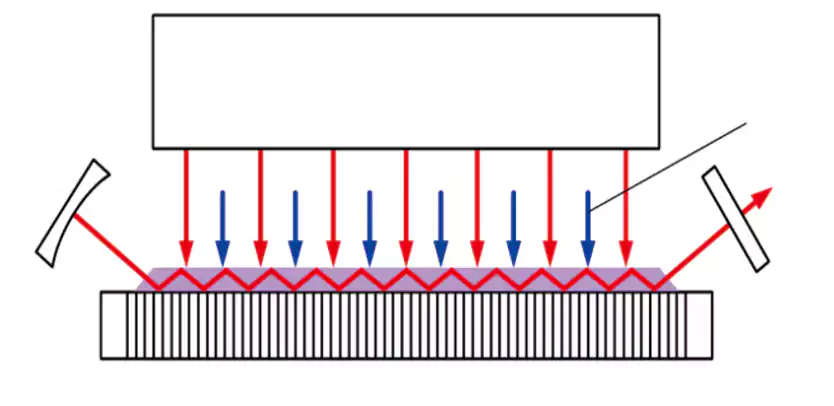
Figure 9. Schematic diagram of experimental optical path
2.Nd:YVO4
Among the gain medium materials, Nd:YVO4 has a large stimulated emission cross-section, five times larger than Nd:YAG, and has an extremely high absorption coefficient for the pump light with a wavelength of 808nm. At the same time, pump absorption is related to polarization. The laser output is linearly polarized along a special direction. The pump absorption of Nd:YVO4 crystal is four times that of Nd:YVO4, avoiding the influence of redundant thermally induced birefringence effects. The gain spectrum line of the Nd:YVO4 crystal is wider and can better adapt to the changes in the pump light wavelength caused by the temperature change of the diode laser. Due to these advantages, Nd:YVO4 is widely used in laser systems that require high efficiency and stability, such as precision measurement, laser marking, medical equipment, spectral analysis, and scientific research experiments. Its ability to deliver high-quality laser output while maintaining low heat losses makes it an integral part of modern laser technology.
Example
1. According to the information published by Fraunhofer Laser Technology in 2003, using Nd:YVO4 crystal with a doping concentration of 0.3% and a size of 1mm×10mm×12mm, a 110w near diffraction limit high beam quality 1064nm TEM00 mode has been obtained Continuous laser output, beam quality factors M2 in two orthogonal directions are 1.3 and 1.5 respectively, light-to-light conversion efficiency is 48%, slope efficiency is 59%, and successfully Q-switched to obtain high power, high beam quality, High repetition rate, narrow pulse laser output.
2. In 2017, Tang Chao and others studied a three-way grazing incidence Nd:YVO4 slab laser amplifier. The schematic diagram of the lath structure is shown in Figure 2. The coordinate origin O is located at the center of the lath, x is the width direction of the lath (width is w), y is the thickness direction of the lath (thickness is h), and z is the length direction of the lath. (length is d). The pumping surface is the front side (area is d×h). The lath is an Nd:YVO4 crystal cut along the c-axis, the doping concentration is 1% (atom number fraction), and the size (d×w ×h) is 20mm×5mm×0.8mm.
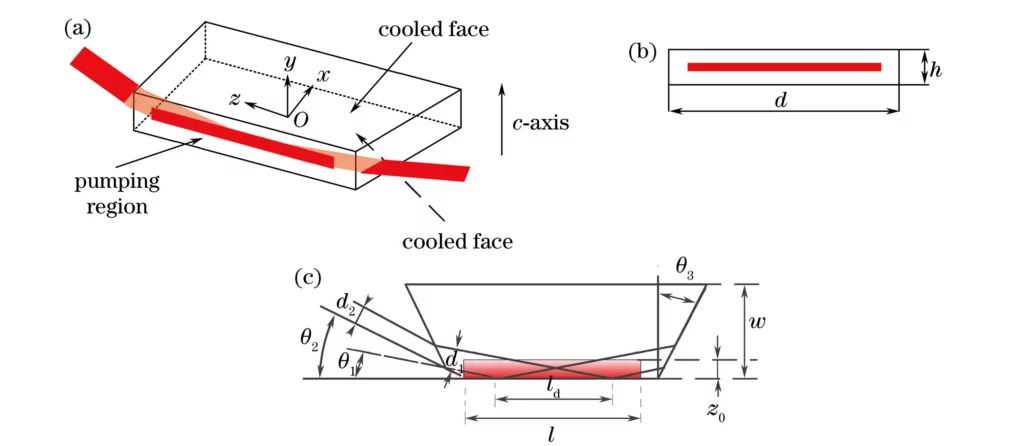
Figure 10. Geometric parameters of Nd∶YVO4 slab laser and pump light. (a) Schematic diagram of the slab structure; (b) Pumping area on the yOz surface; (c) Total reflection of the seed light on the xOz surface on the pumping surface
3.Yb:YAG
Yb:YAG is another popular solid-state laser material favored for its high efficiency and low thermal load. Its main output wavelength is 1030nm, which is particularly suitable for high-power and high-energy slab lasers. Yb:YAG has high thermal conductivity and small thermal effects, which makes it excellent in continuous or high repetition rate pulse operation. With the improvement of diode pumping brightness, Yb:YAG shows greater advantages in high-power laser systems compared to Nd:YAG laser crystals. The energy level structure of Yb:YAG is a quasi-three-level structure (no upper energy level conversion) at room temperature. It has the characteristics of low quantum loss (only 9%), wide absorption spectrum and emission spectrum. Combining these properties, Yb:YAG has become the material of choice in many high-end applications, such as scientific research experiments, industrial processing, medical surgeries, and military equipment used to generate high-power lasers. These advantages make Yb:YAG an important part of modern laser technology and it is expected to continue to play a key role in future technology development.
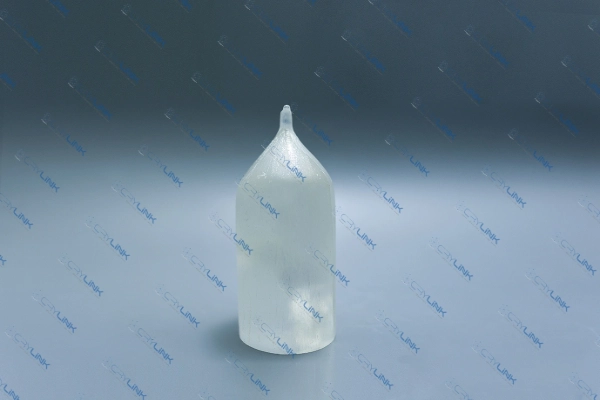
Figure 11.Yb:YAG crystal
Example
In the experiment of 2022, Zhang Fengchen et al., the size of the Yb:YAG slab laser crystal was 1mm × 10mm × 5mm, the doping concentration (atomic number fraction) was 5%, and both end faces of the laser crystal were plated with wavelengths between 1020 and 1060nm. With antireflection coating, the laser crystal is wrapped with indium foil and mounted on a copper block with TEC (ThermoElectricCooler), and the cooling temperature is controlled to 25°C.
Laser Glass
Laser glass consists of matrix glass and activated ions. The various physical and chemical properties of laser glass mainly depend on the matrix glass, and its spectral characteristics are mainly determined by activated ions, but there are also interconnections and influences between them.
Er,Yb∶glass
At present, most eye-safe laser sources in the 1.5 μm band are erbium-doped fiber lasers. However, due to the nonlinear effect in the optical fiber, it is difficult to obtain a larger energy laser output, and its output energy is limited to hundreds of microjoules. Compared with erbium-doped optical fiber media, erbium-ytterbium co-doped phosphate glass is a solid material that can output laser light in the 1.5 μm band, and is expected to obtain high-energy laser output. However, phosphate glass has a large thermal expansion coefficient and a low thermal fracture limit, and is more prone to thermal damage. These are the main reasons that currently limit the output of high-energy lasers by Er, Yb:glass lasers. Compared with the laser medium in the 1μm band, the stimulated emission cross section of Er, Yb:glass in the 1.5μm band is smaller and the thermal effect is serious. Compared with rod-shaped laser media, the slat structure can provide a larger cooling surface.
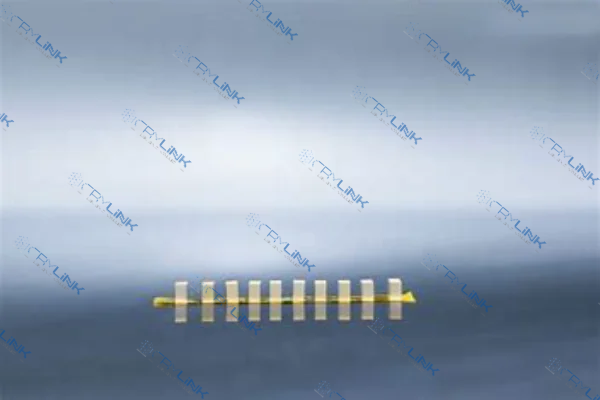
Figure 12. Erbium glass
Example
1. In 2022, Zhang Kexin and others designed an LD array-pumped erbium-ytterbium co-doped phosphate glass (Er, Yb:glass) slab amplifier. Er, Yb: The glass lath structure is as shown in the figure. The length of the lath is 30mm, the width is 5mm, and the thickness is 1mm. The mass fractions of Er3+ and Yb3+ in Er, Yb:glass are determined to be 0.52% and 22%.
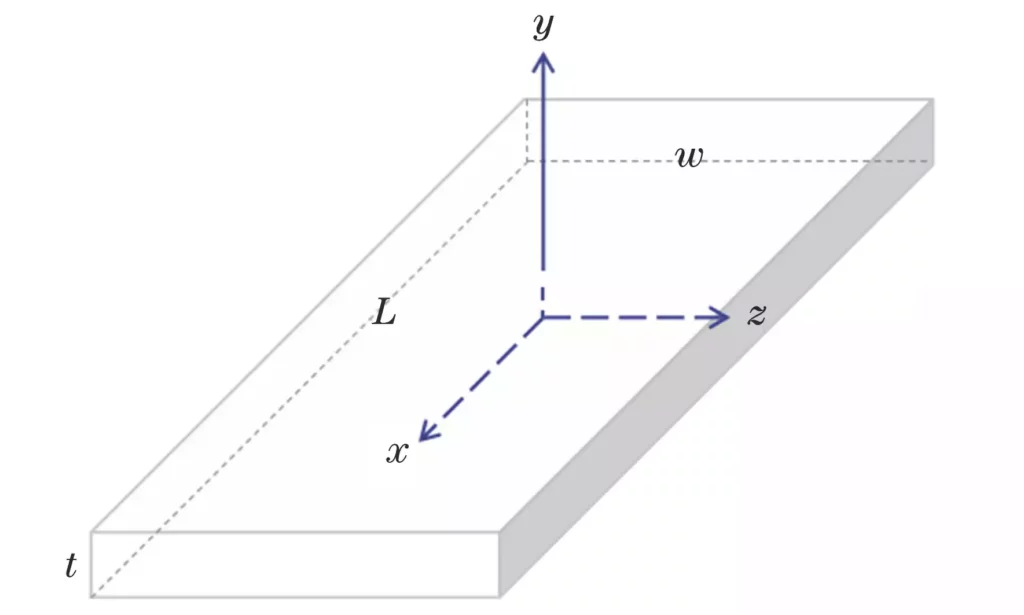
Figure 13.Er,Yb: glass lath structure model
Nd:Glass
Neodymium glass (Nd:Glass) is an important laser material, mainly used to manufacture high-energy lasers. The main feature of this material is an amorphous glass matrix doped with neodymium (Nd³⁺) ions. Since they were first developed in the 1960s, neodymium glass lasers have played an important role in many fields, especially in scientific research, military and medical applications. In addition, it is easy to obtain good optical uniformity in glass, and the shape and size can be freely selected. Large neodymium glass rods are 2m long and 100mm in diameter, which are made into giant pulse energy devices. There are also laminates with a diameter of 90cm and a thickness of 5cm. Small glass fibers with a diameter of only a few microns are used for light in integrated optical circuits. Oscillation and amplification.
Example
1. The laser medium used by Cheng Hongling in 2009 was eight-slat radial neodymium glass. It was cut and processed from neodymium glass rods with a diameter of 30mm. The doping concentration was 3%. One surface was coated with anti-reflection coating, and the other surface was coated with anti-reflection coating. Coated with reflective film with reflectivity >99%, see Figure 6; the size of each slat is 100mm*30mm*4mm
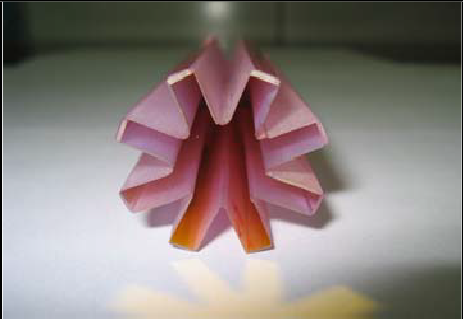
Figure 14. Eight-slat radial neodymium glass
2. In 1995, C.B. Dane and others from Lawrence Livermore National Laboratory in the United States used neodymium glass slabs with dimensions of 14cm × 1cm × 40cm as the amplification medium of the system, and used SBS phase conjugate mirrors to correct wavefront distortion, and obtained The pulse energy is 25-30J, the pulse width is 14ns, and the average output power is 150W, near the diffraction limit output.
3. In 1997, Milton J. and others from the University of Rochester in the United States reported a Brewster’s angle neodymium glass slab amplifier with a slab size of 31.0cm×8.0cm×1.1cm.
4. In 2008, RyoYasuhara and others from Osaka University in Japan reported their high pulse energy and high peak power laser system. The average output power of the system is 213W, the repetition frequency is 10Hz, the pulse width is 8.9ns, and the peak value reaches 2.4GW. Its system structure is shown in Figure 7. The main amplifier of the system is a neodymium glass slab pumped by two laser diodes, with the dimensions of the slab being 5cm × 1cm × 37.5cm.
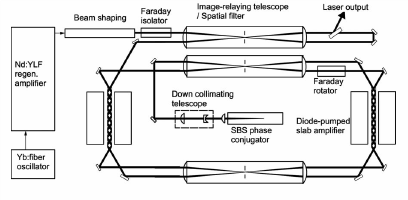
Figure 15. Diode-pumped neodymium glass slab laser system using TECS technology and SBS phase conjugate mirror
Bonded Crystal
1. YVO4+Nd:YVO4+YVO4
The thermal conductivity of Nd:YVO4 is 11.7W/cm/°K@25°C, the thermal conductivity of pure YVO4 crystal is //C:5.23W/m/K; ⊥C:5.10W/m/K, both End bonding to form a YVO4+Nd:YVO4+YVO4 bonded crystal can effectively improve the thermal effect of the Nd:YVO4 crystal, reduce the thermal lens effect formed during laser pumping, improve the laser beam quality, and increase the 457nm and 671nm laser output efficiency. It can also improve the output stability of the laser and extend the service life of the laser.
Example
1. In 2023, Song Yue et al. proposed an electro-optical Q-switched laser with high beam quality, narrow nanosecond pulse width, and high repetition frequency pulse train output. By optimizing the aspect ratio of the doped region of the bonded Nd:YVO4 slab crystal, combined with the optimal design of the cavity mode, to limit the high-order mode oscillation in the cavity, high-beam quality laser output similar in two directions was obtained. The lath crystal used is YVO4/Nd:YVO4/YVO4 bonded crystal, and the crystal structure is shown in Figure 16.
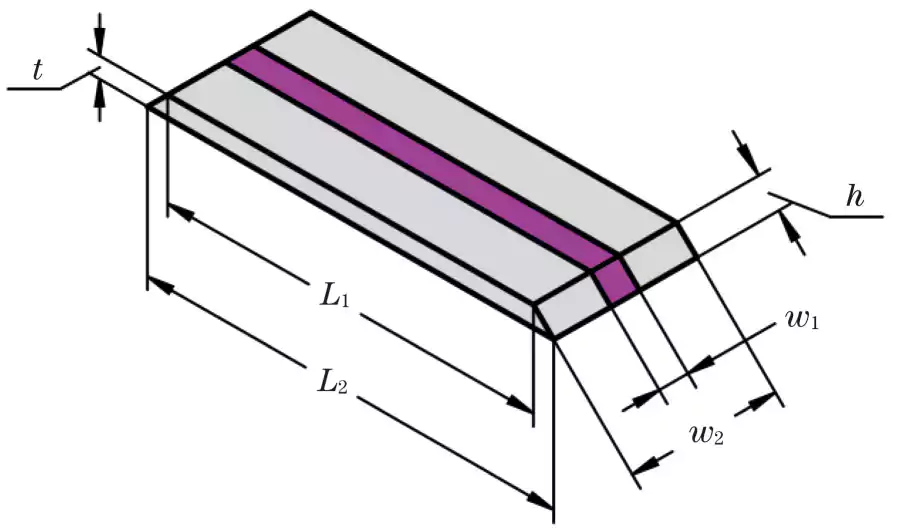
Figure 16. Schematic diagram of the geometric structure of Nd∶YVO4 lath crystal
The length L2 of the large surface of the crystal is 12.78mm, the side length L1 of the large surface is 11.36mm, w2 is 5mm, w1=h=1.0mm, and the thickness t is 0.71mm.
The Nd∶YVO4 crystal is cut along the a-axis. The atomic fraction of doped Nd3+ is 2%. Undoped YVO4 crystals with a width of 2mm are bonded on both sides of the doped area. The purpose is to increase the heat dissipation area of the lath crystal and constrain the pump. Pu area.
Slat Laser Crystal Material Manufacturer
The following are ranked in no particular order
1、Crylink
Crylink is a company focusing on the field of optoelectronics, mainly engaged in the research and development, production and sales of laser crystals, nonlinear optical crystals, laser components and related optoelectronic products. Their products are widely used in laser technology, medical care, biology, optical communications, industrial testing and other fields.
Crylink’s advantage lies in its strong R&D capabilities and advanced production technology. The company has a team of professionals who are committed to improving product performance and reliability through innovation. Crylink’s product lines range from basic laser crystals to complex optical components, including but not limited to various types of nonlinear optical crystals, laser crystals and photoelectric detection devices. They are able to design and manufacture customized products according to customers’ specific needs to meet the requirements of different application scenarios. This customer-oriented approach enables Crylink to remain competitive in the optoelectronics industry and provide customers with high-quality products and services.
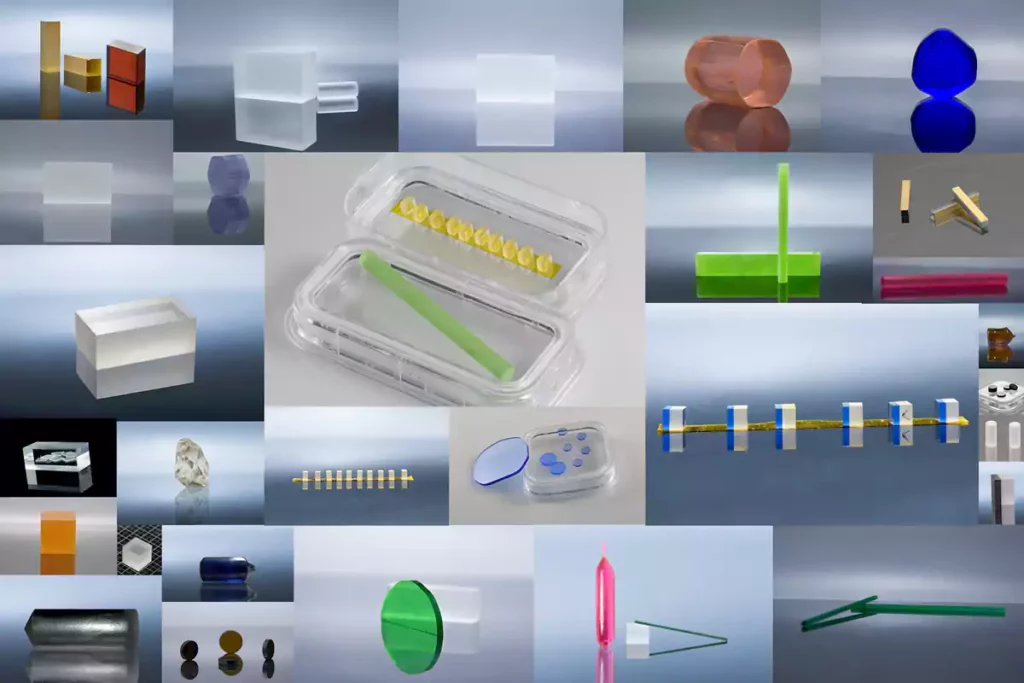
Figure 17. Crylink product assembly diagram
2、EKSMAOptics
EKSMA Optics manufactures precision optical laser components for use in lasers and other photonic instruments and has extensive experience in providing customized solutions and offering a variety of catalog products for fast, off-the-shelf delivery. The product range includes laser optics, DKDP, BBO and RTP Pockels cells with high voltage drivers, laser media and nonlinear crystals, optomechanical mounts, ultrafast pulse selectors.
EKSMAOptics polishing equipment specializes in polishing flat optics made of BK7, UVFS, Infrasil, Suprasil, DKDP, LBO and ZnGeP2 crystals for high power laser applications. The company also has a lens manufacturing department for the production of precision spherical and aspheric lenses and a coating deposition department based on IBS technology.
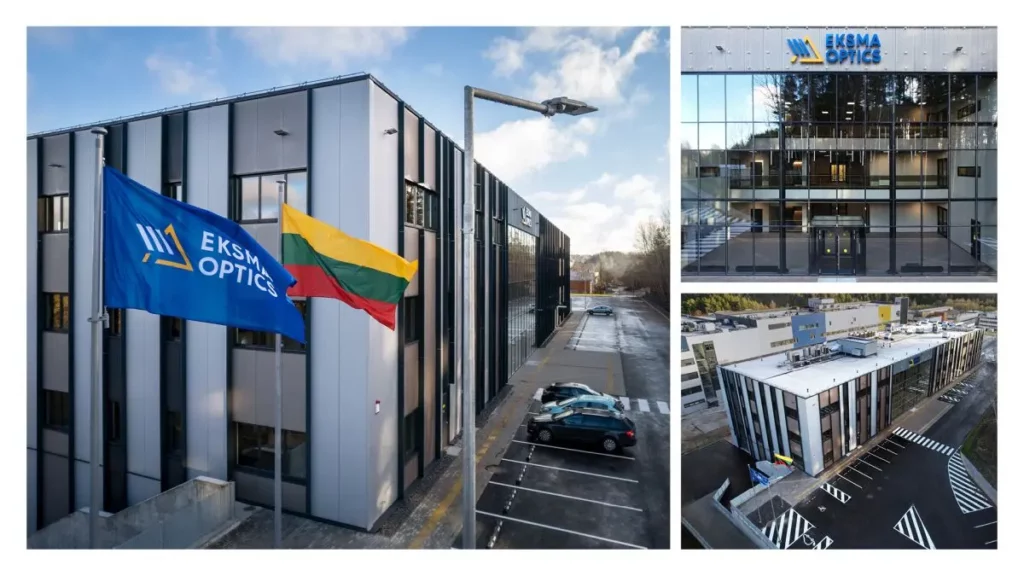
Figure 18.EKSMAOptics
3、Optogama
Optogama was founded in 2015 and is located in Lithuania. is a high-tech company that designs, develops and commercializes custom and unique laser products. We develop technologies for laser applications in analytical and spectroscopic instrumentation, automotive, sensing, medical and materials processing. With a clean room environment, in-house CNC machining capabilities and over 15 years of experience in the laser and photonics industry, high quality innovative products are produced.
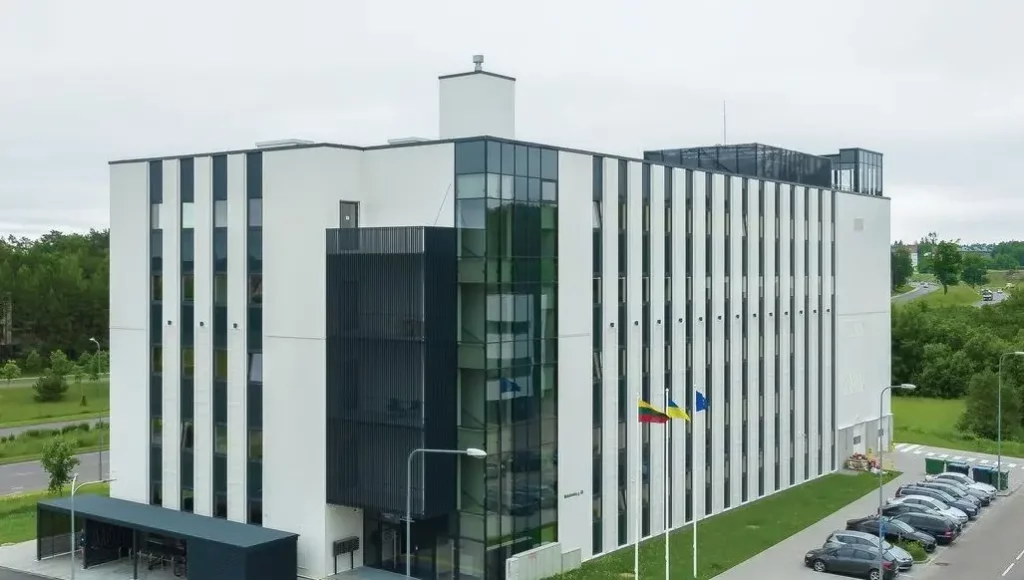
Figure 18.Optogama
4、CASTECH
In the rapid development of science and technology, CASTECH has become a leading enterprise in the optoelectronics industry with its innovative spirit and forward-looking thinking. We focus on the R&D and manufacturing of high-end optoelectronic products and are committed to providing customers with the highest quality solutions and services. CASTECH’s product lines cover a wide range from advanced laser devices to precision optical components, involving many fields such as communications, medical care, and industrial processing.
The core advantage of CASTECH lies in continuous technological innovation and strict quality control. Through close cooperation with top scientific research institutions at home and abroad, CASTECH continues to push the boundaries of optoelectronic technology, aiming to provide products with higher efficiency and lower energy consumption. Our R&D team is composed of senior experts and young innovative talents in the industry, working together to develop internationally competitive high-tech optoelectronic products.
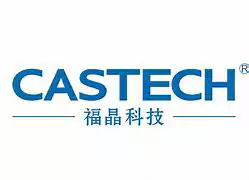
Figure 20.CASTECH logo
5、ALPHALASGmbH
ALPHALASGmbH is a laser manufacturer founded in 1997 in Göttingen, Germany. The main business includes the development, production and sales of lasers, laser systems, optical devices, laser-related electronic products and components. The main products are advanced diode-pumped solid-state lasers, single-frequency and sub-nanosecond Q-switched lasers. In addition, laser crystals such as Nd:YAG, Cr:YAG, Yb:YAG, Cr4+:YAG, Nd3+:YAG+V3+:YAG, Er3+:YAG+YAG, Nd3+:YVO4+YVO4 are available from stock, and can also be customized according to AR, PR or HR coating available to customer specifications.
6、Laserton
Laserton is a manufacturer specializing in the production of laser crystals, nonlinear crystals and various precision optical components. Large-diameter laser crystals can be grown using Czochralski technology and can provide Nd:YAG, Ce:YAG, Cr4+:YAG, Er:YAG, Nd:YVO4, Yb:YVO4 and other laser crystals. Our optical crystals have excellent optical uniformity. We can provide BBO, KTA, KDP, LBO, SBN, KDP, LiNbO3 nonlinear crystals. In addition, we offer electro-optical KTP and RTP crystals with high damage threshold and low half-wave voltage for Q-switching applications.
References:
[1] Wang Huihua, Lin Longxin, Ye Xin. Current status and development trends of high-power slab laser technology [J]. Infrared and Laser Engineering, 2020. DOI: 10.3788/IRLA20190456.
[2] Yang He. Research on high-power slab laser oscillators and amplifiers [D]. Shandong University, 2013.
[3] Zhao Haichuan. Research on multi-angle amplification of end-pumped Zig-Zag slab laser media [D]. National University of Defense Technology, 2010. DOI: CNKI: CDMD: 2.2009.213946.
[4] Shi Bingnan. Theoretical and experimental research on solid ultrafast laser amplification [D]. Shandong University, 2022.DOI:10.27272/d.cnki.gshdu.2022.000495
[5] Li Shimin, Huang Weiling, et al. Principle and design of laser devices. 2nd edition. National Defense Industry Press, 2005: 170~171;235~239
[6]KoechnerW.SolidStateLaserEngineering[M].6thed.Berlin:Springer,2006.
[7]MartinWS,ChernochJP.Multipleinternalreflectionfacepumpedlaser:USPatent,3633126[P].1972-12-05.
[8]RutherfordTS,TullochWM,GustafsonEK,etal.Edge-pumpedquasithreelevelslablasers:designandpowerscaling[J].IEEEJQuantumElectron,2000,36(2):205−219.
[9] Li Jinping. Theoretical study on thermal effects of slab laser crystals [D]. Xi’an University of Architecture and Technology, 2012. DOI: CNKI: CDMD: 2.1011.297059.
[10] Zhang Xinghu, Tu Wei, Chen Baizhong, et al. All-solid-state high-power quasi-continuous slab laser [J]. Acta Luminescence, 2023, 44(04): 673-677. DOI: 10.37188/CJL.20220352.
[11] Tang Chao, Xu Shuangfu, Zhang Xiang, Wang Yong, Liu Bin, Liu Dong, Xiang Zhen, Ye Zhibin, Liu Chong. Optimized design of grazing-incidence Nd∶YVO4 slab laser amplifier [J]. China Laser, 2017, 44( 12):1201003.
[12] Gao Qingsong, Zhou Tangjian, Shang Jianli, et al. Research on efficient and compact room temperature Yb:YAG slab all-solid-state laser technology [J]. Intense Laser and Particle Beam, 2020, 32: 121009.doi:10.11884/HPLPB202032.200185
[13] Fengchen Zhang, Chenlin Hui, Danli Wang, Honglin Liao, Zhili Lin, Shaojian Su, Fengjiang Zhuang. Polarization Control in Passively Q-Switched Dual-Wavelength Yb∶YAG Slab Laser[J]. Acta Optica Sinica, 2022, 42(8): 0814003.
[14] Zhang Kexin, Wang Kaixin, Gao Chunqing. Design and thermal effect analysis of LD pumped Er, Yb: glass slab multi-pass amplifier [J]. Acta Optica Sinica, 2022, 42(5):0514001.
[15] Cheng Hongling. Preliminary study on the theory and experiment of radiating slab solid-state laser [D]. Harbin Institute of Technology, 2011.
[16] Xiao Ligang. Analysis of thermal effects of laser medium on large-size neodymium glass slabs [D]. Beijing University of Technology, 2010.
[17] Yue Song, Zhimin Wang, Xihang Yang, Yong Bo, Fengfeng Zhang, Yixuan Zhang, Zihan Zhou, Wencheng Zhao, Yanyong Lin, Li Fu, Qinjun Peng. Nd∶YVO4 Slab Burst‐mode Laser with High Beam Quality and Short Nanosecond Pulse Width[J]. Chinese Journal of Lasers, 2023, 50(7): 0701004.
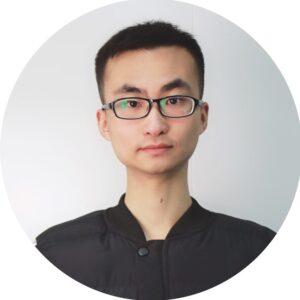
Frank
Frank graduated from the University of Shanghai for Science and Technology, majoring in optics. As a technical engineer at Crylink Company, he deeply understands crystal materials and laser components.
Related Video(s) with this Article
Related Product(s) with this Article
Related Application(s) with this Article